摘要
深水湖泊冬季热力分层消亡,水体上下混合,水动力过程改变营养盐的分布格局,可能影响湖泊生态系统中浮游植物群落的演替。本研究在2022年11月—2023年4月高原深水湖泊抚仙湖的热力分层消亡期、混合期和分层形成期,开展水体垂直剖面的理化环境因子及浮游植物群落高频观测,探究浮游植物群落时空变化特征及其对热力学混合过程的响应。结果表明:水体混合过程引起湖泊理化环境因子显著的时空变化,与分层消亡期和形成期相比,混合期水体总磷(TP)、溶解性有机磷(DOP)、正磷酸盐(PO3-4-P)和叶绿素a(Chl.a)浓度显著增加,总氮(TN)、溶解氮(DN)、颗粒氮(PN)、氨氮(NH3-N)和硝态氮(NO-3-N)浓度显著降低。浮游植物群落中,绿藻门的转板藻属(Mougeotia)和小球藻属(Chlorella)在整个观测期密度较高,蓝藻门的长孢藻属(Dolichospermum)和拟柱孢藻属(Cylindrospermopsis)在分层消亡期密度较高,硅藻门的直链藻属(Melosira)和隐藻门隐藻属(Cryptomonas)在混合期密度高,绿藻门的栅藻属(Scenedesmus)在分层形成期密度较高。冗余分析发现,NH3-N、PO3-4-P和碱性磷酸酶活性的动态变化显著影响浮游植物群落组成;而且,浮游植物不同种类对氮、磷营养盐的敏感性各不相同,其中长孢藻属和转板藻属的密度与氮营养盐呈正相关、与磷营养盐呈负相关;而其他浮游植物密度则呈现相反规律,分别与氮营养盐呈负相关,与磷营养盐呈正相关。通过结构方程模型分析水体混合期间浮游植物群落动态变化的驱动机制,发现一方面水温对浮游植物群落结构有直接影响;另一方面,水温通过调节不同形态的氮(NO-3-N、NH3-N)、磷(PO3-4-P和DOP)营养盐浓度,从而间接地驱动浮游植物群落结构变化。这些直接和间接的影响共同作用,最终引起水体中Chl.a浓度变化。本研究有助于认识深水湖泊热力分层变化对生态系统结构和功能的影响。在全球变化背景下,加强对湖库热力分层的作用机制及其生态环境效应研究,有助于深水湖泊生态系统的保护和管理。
Abstract
During winter, the decline of thermal stratification and the mixing of water in deep lakes can alter the distribution pattern of nutrients, and potentially affect the succession of phytoplankton communities in lake ecosystems. In this study, high-frequency observations of physicochemical environmental factors and phytoplankton communities were conducted along vertical profiles during stratification decline, mixing, and stratification formation periods in Lake Fuxian, a deep plateau lake, from November 2022 to April 2023. The spatiotemporal characteristics of phytoplankton communities in response to the thermodynamic mixing process were investigated. The results showed that the mixing process caused significant spatiotemporal changes in physicochemical environmental parameters. Compared with the stratification-declining period and stratification-formation period, the concentrations of total phosphorus (TP), dissolved organic phosphorus (DOP), phosphate (PO3-4-P), and chlorophyll-a (Chl.a) in the water were significantly increased during the mixing period, while the concentrations of total nitrogen (TN), dissolved nitrogen (DN), particulate nitrogen (PN), ammonium nitrogen (NH3-N), and nitrate nitrogen (NO-3-N) were significantly decreased by mixing. Among the phytoplankton community, the green algae Mougeotia and Chlorella had higher densities than other species throughout the observation period, while the cyanobacteria Dolichospermum and Cylindrospermopsis had the highest densities during the stratification-declining period, and the diatom Melosira and the cryptomonad Cryptomonas had the highest densities during the mixing period, and the green algae Scenedesmus had the highest densities during the stratification formation period. Redundancy analysis showed that the dynamic changes in NH3-N, PO3-4-P, and alkaline phosphatase activities significantly influenced the composition of the phytoplankton community. Moreover, the sensitivity of phytoplankton to nitrogen and phosphorus nutrients varied among different species. Dolichospermum and Mougeotia showed a positive correlation with N nutrients and a negative correlation with nutrients, while the densities of other phytoplankton species exhibited an opposite pattern, showing a negative correlation with nitrogen and a positive correlation with phosphorus. Structural equation modeling was used to analyze the driving mechanisms of phytoplankton community dynamics during the water mixing period. On one hand, water temperature directly impacted the structure of the phytoplankton community. On the other hand, water temperature indirectly drove changes in the structure of the phytoplankton community by regulating the concentrations of different forms of nitrogen (NO-3-N, NH3-N) and phosphorus (PO3-4-P and dissolved organic phosphorus). Ultimately, these direct and indirect effects collectively contribute to the changed in the concentration of Chl.a. This study contributed to our understanding of the effects of thermal stratification changes on the structure and function of lake ecosystems in deep lakes. In the context of global changes, strengthening research on mechanisms and ecological environmental effects of thermal stratification in lakes and reservoirs can support the protection and management of lake ecosystems.
Keywords
水体热分层是由于季节性温度变化引起的一种自然现象,它在全球的自然湖泊中普遍存在,其形成主要受到水深、湖泊形态及气候条件等因素的影响[1-2]。水深是湖泊热分层形成的重要条件之一[3],浅水湖泊一般较难形成温跃层,因此热分层常出现在深水湖泊[4-6]。在高温季节,深水湖泊上层湖体由于受到气温及热对流等因素影响,上、下层产生温差[6-7],引起湖体水密度产生差异[8],进而导致垂向剖面自上而下形成变温层、温跃层和恒温层[9]。稳定的热分层会阻碍上、下层水体的交换,导致沉积物形成缺氧环境[10-11],而这种缺氧环境会促使内源物质释放并在恒温层中积累 [12]。此外,在温跃层上、下会形成“热/冷”“氧化/还原”的对立环境[12-14],进而导致深水湖泊的物理、化学性质和初级生产力都产生显著差异[15]。Dresti等[16]在1993—2020年对意大利/瑞士Maggiore湖的研究发现,温度升高增强了湖泊热分层,导致湖泊溶解氧(DO)消耗增加,进而限制了营养物质从底层向上层的输送和补充。Sun等[17]对潘家口水库水体及沉积物的研究发现,在热分层期底层缺氧条件下,沉积物中磷主要以有机物磷和铁磷的形式释放。Zhang等[18]在对千岛湖湖水热分层的高频监测研究中发现,千岛湖在春季热分层期间浮游植物主要聚集在水体上层。
但随着季节性气温骤降,水体垂向剖面温差减小,热分层逐渐消失,并在一段时间内上下层水体发生垂向对流混合现象,这期间为水体混合期[19]。热分层期间,在恒温层中积累的营养盐和浮游生物等会在温跃层消失后,通过水体的垂直对流作用向上层水体迁移,并对湖泊的生态环境产生直接影响。因此,在混合期,水体的理化指标和初级生产力成为影响湖泊生态环境的重要因素。而浮游植物作为湖泊的主要初级生产者,它的初级生产力是评价湖泊生态系统能量生产效率和生物量生成能力的关键指标,揭示了湖泊中能量的产生、转化和流动过程[20-21],而湖泊热分层“起止”这一物候变化对浮游植物的初级生产力产生的显著影响主要体现在两个方面:一方面是直接影响发生光合作用的营养生成层范围[15];另一方面是通过影响深水对表层水体的营养供应,进而间接对浮游植物群落结构产生影响[22-23]。目前,关于湖泊阶段性或周期性水体混合事件的研究已经得到了广泛关注,多个国家和地区的湖泊,例如中国的贵州百花湖水库[4]、南宁天雹水库[24],土耳其的Tahtali水库[25],以及印度西部的Tillari水库[26]等都有相关报道。然而,相较于水体混合现象本身的研究,关于浮游植物群落如何对这些水体混合过程做出响应的研究还相对较少。
抚仙湖是我国重要淡水湖泊之一,目前对其水体热分层的研究主要集中在夏季热分层期间水温分层的水深特征、对理化性质的影响以及浮游植物的分布情况[6,27-28],但对冬、春季水体混合期间的理化性质及垂向剖面的浮游植物群落结构变化及影响因子则较少涉及。而且,近几十年来,在人类活动引起的营养盐输入增加、气候变化、水体热分层特征的变化、外来物种的引入以及湖泊富营养化等多种因素共同作用下[28-29],抚仙湖浮游植物群落结构主要由清水性种类向喜营养种类转变[30-31]。蒋伊能等[32]对抚仙湖浮游植物的调查发现,冬、春季优势种属主要包括绿藻门的转板藻(Mougeotia)、斜生栅藻(Scenedesmus obliquus)和四角藻(Tetraedrom),硅藻门的小环藻(Cyclotella)和克洛脆杆藻(Fragilaria crotomensis),蓝藻门的棒胶藻(Shabdogloea)以及隐藻门的隐藻(Cryptomonas)和蓝隐藻(Guillardia)。考虑到热分层周期变化所带来的物理扰动可能会影响沉积物中内源磷的释放,因此迫切需要对抚仙湖进行研究,以了解水体分层和混合如何影响营养盐等理化因素,以及浮游植物群落如何对此作出响应。
为了解抚仙湖水体混合对浮游植物时空变化的影响及机理,本研究对水温进行垂向剖面高频原位监测,分析了抚仙湖水体热分层结构形成、稳定和消亡的演变过程;并通过定点垂向剖面采样,在揭示水体混合期间垂向剖面的理化性质和浮游植物群落的时空变化特征的基础上,探究水体混合期间浮游植物变化的关键驱动因子,以期为保护抚仙湖生态安全提供科学依据,为高原深水湖泊的进一步研究提供科学参考。
1 材料与方法
1.1 研究区域概况
抚仙湖位于云南省玉溪市澄江县、江川县、华宁县之间,是我国第二深水湖泊,属珠江流域南盘江水系[6]。抚仙湖受构造盆地影响,湖泊形态主要呈南北向分布,南北长31.5 km,东西宽11.5 km,湖泊面积212 km2,平均水深95.2 m,最大水深近155 m[33]。抚仙湖处于亚热带半湿润季风气候区,受季节变化影响抚仙湖水温具有显著季节差异[6]。为探究抚仙湖在热分层消亡过程中水体变化特征,本研究于2022年11月—2023年4月进行水体高频监测,高频监测的数据来自抚仙湖北部湖区布设的固定浮标(24°31′29″N,102°56′9″E),该位置海拔1721 m,水深约60 m,与湖岸线最小距离约280 m,受入湖径流影响较小。
1.2 水温高频观测和样品采集
为了精确监测水体温度变化,沿固定浮标从水面至60 m水深处,每2 m深度设置一个高精度的水下温度记录仪(HoBo Onset MX2203),总计布设30个。记录仪以每小时一次的频率连续记录水温(WT)。于2022年1月—2023年4月对水温进行在线垂向监测及分析。同时于2022年11月—2023年4月在固定浮标上每隔10天进行垂向水体分层采样,共计采样15次。按照温度垂向梯度≥0.20℃/m定义温跃层[34-35],变温层和温跃层水温变化较大,而恒温层水温变化较小,所以从表层到50 m每间隔5 m采集一个水样,50 m以下每隔10 m采集一个水样,每次获得12个分层样品。
1.3 热分层稳定指数
(1)
(2)
式中,ρi为不同深度处的水体密度(kg/m3);Ti为水体不同深度处的温度(℃),由各水深处的Ti便可求出此处的ρi,从而得到水体密度梯度;Db为底部(底泥上0.2 m)水体密度(kg/m3);Da为表层(水下0.5 m)水体密度(kg/m3);D4、D5分别为4、5℃条件下纯水的密度(kg/m3);H为水深(m)。
1.4 理化指标测定
总氮(TN)、溶解氮(DN)、总磷(TP)、溶解磷(DP)、氨氮(NH3-N)、硝态氮(NO-3-N)、亚硝态氮(NO-2-N)、正磷酸盐(PO3-4-P)、溶解性有机碳(DOC)、叶绿素a(Chl.a)、碱性磷酸酶活性(alkaline phosphatase activities,APA)等各项水体理化指标按照《地表水和污水监测技术规范》[38]测定。为保证指标数据的质量,每次样品均设置阳性对照来监控不同批次样品数据。颗粒态氮(PN)、颗粒态磷(PP)和溶解性有机磷(DOP)的质量浓度计算公式分别为:
(3)
(4)
(5)
式中,ρDIP为溶解性无机磷浓度。
叶绿素a(Chl.a)是监测水体中浮游生物现存量的常用指标,在一定程度上也可体现初级生产量的大小[39-40]。本研究取1 L水样通过玻璃纤维GF/C滤膜过滤,对折装至15 mL离心管中,然后加入10 mL体积分数为90%的丙酮溶液萃取Chl.a,在4℃避光条件下保存24 h,期间摇晃4~5次,使其充分萃取。最后用分光光度仪分别在波长630、645、663、750 nm下测定吸光度值,计算公式为:
(6)
式中,Chl.a为水样中叶绿素a浓度(mg/m3或者μg/L),D630、D645、D663、D750分别为波长630、645、663、750 nm处的吸光度值,V1为萃取液体积(mL),V为水样体积(L),ε为比色皿光程(cm)。
碱性磷酸酶可在环境中催化DOP转化为DIP供生物直接利用[41],其酶活性在一定程度上可表征环境中生物可利用的DIP是否充足。因此本研究以10 mg/L对硝基苯磷酸二钠作为底物,以硝基苯酚的产生速率作为APA的指标。测定步骤为:取水样150 μL,用Tris HCl缓冲溶液调pH 值为8.4,加150 μL底物,在30℃下保持6 h;按上述方法,以去离子水代替水样做空白对照;分别以0、30、60、90、120、150、180 μL的10 mg/L对硝基苯酚与去离子水添加至总体积为300 μL作为标准曲线;然后用分光光度仪在波长410 nm下测定[41],计算公式为:
(7)
式中,Λ(APA)为碱性磷酸酶活性(nmol/(mL·h)),n为水解反应后产生的对硝基苯酚的量(nmol),V为水样的体积(μL),t为反应时间(h)。
1.5 浮游植物鉴定以及群落Beta多样性贡献指数
取1 L水样,加入鲁哥试剂后避光带回实验室,用于浮游植物鉴定。水样静置96 h,吸去上清液,收集30 mL浓缩样品,完全混合后于0.1 mL的计数框中在显微镜下计数,每个样品计数3次,若3次计数结果与其均值之差大于15%,则进行第4次计数。浮游植物物种鉴定主要参照《中国淡水藻类——系统、分类及生态》[42],计算公式为:
(8)
通过计算物种分布矩阵Y中每行的方差(SSj)占总方差(SStotal)的比重可获得各样品中Beta多样性的贡献指数(local contribution to Beta diversity,LCBD)[43]。具有高LCBD数值的位点往往具有不同或特有的物种组成[44]。对于由n个位点和p个物种构成的物种分布矩阵,LCBD的计算方法为:
(9)
式中,yij为物种i在群落j中的多度,为物种i在全部群落中的均值。
1.6 数据统计分析
采用SPSS 27软件计算均值、标准差并进行单因素方差分析(P<0.05),采用Origin 2021进行相关性聚类分析[45],采用CANOCO5进行冗余分析。
结构方程模型(structural equation modeling,SEM)既包含显变量又有潜变量[46-48],不仅可以通过通径分析探究变量之间的关系,还可以解释变量的变异或变量之间的共变(variation and covariation)关系[47]。为进一步探索相关环境因子对浮游植物变化的影响机理以及这些关键因子之间的相互作用关系,本研究采用SmartPLS 3绘制结构方程模型(P<0.05),利用偏最小二乘回归法(partial least squares regression,PLS算法)对浮游植物聚类结果和环境因子进行通径分析,用规范拟合指数(normed fit index,NFI)判定模型拟合程度[48]。
2 结果
2.1 水质理化指标时空分布特征
2.1.1 水温时空变化特征
抚仙湖2022年1月—2023年4月水温在13.70~26.34℃之间变化,最高、最低水温分别出现在8月表层水体和2月60 m深度处水体(图1A)。水体分层期间:①表层至水深15 m为变温层,水温在22~26.34℃之间,且垂向分布较均匀;②水深15~40 m为温跃层,水温垂直梯度平均达0.23℃/m;③水深40 m以下为恒温层,水温逐渐趋于平稳并恒定至(14.31±0.58)℃。
抚仙湖的水温监测数据表明,湖泊在一年内会经历一次明显的热分层和混合周期。即便在冬季气温较低时,湖体水温也没有出现逆分层现象。这说明抚仙湖的水体热分层特征符合典型的单循环混合模式。根据水温数据计算RWCS和温跃层出现及消失时间,可以将全年变化划分为4个阶段[21](图1B):①分层形成期:从3月中旬到4月中旬,水温垂向变化范围为13.73~19.22℃,温跃层开始出现,但此时RWCS在(1.00±0.51)~(1.69±0.55)m-1之间,不足以形成稳定分层;②层化期:水温垂向差异增大,温跃层稳定,4月中旬后RWCS由(1.00±0.51)m-1增至(7.74±0.85)m-1,热分层稳定状态在4—11月上旬得以保持;③热分层消亡期:11月中旬开始,水温垂向变化范围为14.22~19.94℃,温跃层逐渐消失,RWCS在(1.00±0.51)~(1.69±0.55)m-1之间;④混合期:12月中旬温跃层完全消失,RWCS在(1.00±0.51)m-1以下开始完全混合,直到次年3月,水体基本处于同温混合状态。
2.1.2 水质化学指标响应特征
根据RWCS,将水质指标采样周期(2022年11月—2023年4月底)分为热分层消亡期(2022年11月7日—2022年12月10日)、混合期(2022年12月18日—2023年3月17日)和热分层形成期(2023年3月27日—2023年4月27日)。在3个时期,从表层到60 m水深的平均WT呈现显著差异:分层消亡期>分层形成期>混合期(图2A)。从表层到60 m水深的氮、磷营养盐平均浓度和其他化学指标也呈现一定的时空响应特征。3个时期中,除TN、DN、NO-2-N外, PN、NH3-N和NO-3-N等氮营养盐平均浓度均在混合期最低(图2B~G);除PP外,TP、DP、PO3-4-P和DOP等磷营养盐平均浓度均在混合期最高(图2H~L);DOC平均浓度在混合期最低,与分层消亡期差异显著(图2M);水体Chl.a平均浓度在混合期最高,但与分层形成期不存在显著差异(图2N);APA在分层消亡期最低,在分层形成期最高,3个时期差异显著(图2O)。这表明在混合期抚仙湖大部分氮、磷营养盐浓度处于降低或者升高的动态过程中。
2.2 浮游植物群落时空变化特征
抚仙湖蓝藻门密度大小依次为长孢藻属(Dolichospermum)>假鱼腥藻属(Pseudoanabaena)>拟柱孢藻属(Cylindrospermopsis)>平裂藻属(Merismopedia);绿藻门密度大小依次为小球藻属(Chlorella)>转板藻属(Mougeotia)>栅藻属(Scenedesmus)>纤维藻属(Ankistrodesmus);硅藻门密度大小依次为直链藻属(Melosira)>脆杆藻属(Fragilaria)和隐藻属(Cryptomonas)(图3)。除了小球藻属,绝大多数浮游植物以浅水层分布为主(105 cells/L藻密度集中在20 m水深以上),深水层分布减少,密度呈明显的垂直分布模式。在时间上:① 长孢藻属、小球藻属和假鱼腥藻属在分层消亡期占优势,其中长孢藻属密度最高,达5×105 cells/L;②小球藻属和转板藻属在混合期占据优势,分别在10~20 m、50 m水层出现最高密度(8×105和3.34×105 cells/L);而长孢藻属、拟柱孢藻属和假鱼腥藻属未在整个混合期持续占据优势。③ 小球藻属、转板藻属和平裂藻属在分层形成期占优势,藻密度最高分别为8×105、3.34×105和6.56×103 cells/L(图4)。浮游植物群落LCBD在分层消亡期、混合期和分层形成期分别为0.0042±0.0026、0.0062±0.0039和0.0049±0.0042,混合期LCBD显著高于分层消亡期和分层形成期。
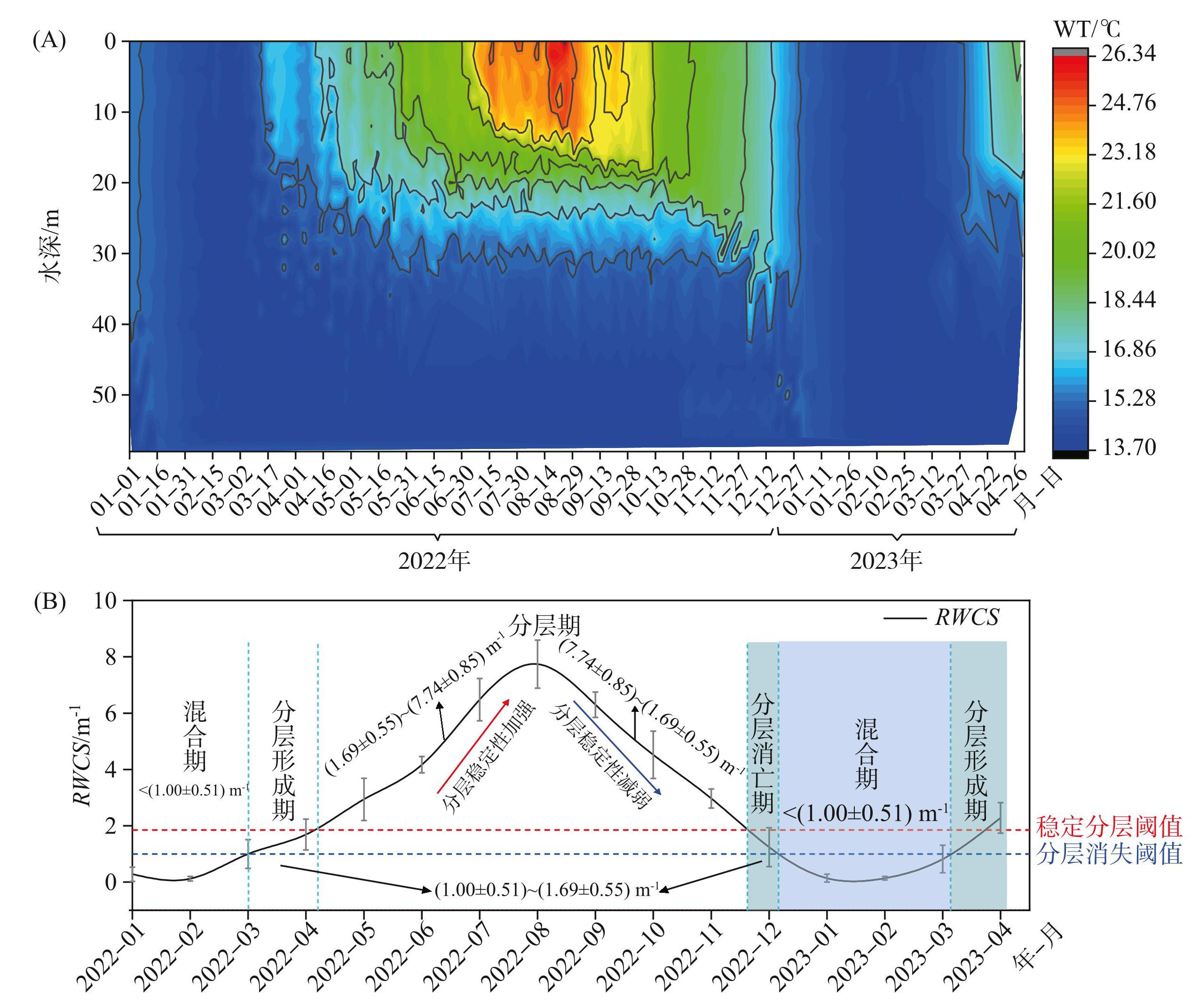
图1抚仙湖(A)垂向水温和(B)热分层稳定指数变化(图B不同背景颜色表示不同水质指标采样时期)
Fig.1(A) Vertical water temperature and (B) relative water column stability of Lake Fuxian over time (The colared areas in Figure B indicate three periods of observation)
2.3 环境因子与浮游植物的相关性分析
2.3.1 抚仙湖水体混合前后影响浮游植物变化的关键因子
将3个时期的环境因子去冗余后分别与对应时期的浮游植物进行冗余分析(RDA):①分层消亡期,轴1和轴2分别解释了13.51%和2.21%的藻类群落组成变化;假鱼腥藻属、拟柱孢藻属、长孢藻属和转板藻属主要受到DN和NH3-N的影响,而直链藻属和纤维藻属与NH3-N的相关性较弱(图5A)。②混合期,轴1和轴2分别解释了19.22%和6.44%的藻类群落组成变化;拟柱孢藻属、长孢藻属和假鱼腥藻属主要受到WT和NH3-N的影响;而其他藻类主要受到PO3-4-P、Chl.a和APA的影响(图5B)。③分层形成期,轴1和轴2分别解释了19.06%和6.54%的藻类群落结构变化;假鱼腥藻属、长孢藻属和脆杆藻属主要受到NH3-N和DOC的影响,转板藻属、隐藻属和栅藻属主要受到PO3-4-P的影响(图5C)。
2.3.2 抚仙湖水体混合期间浮游植物时空变化的主要驱动因子及影响机理
将浮游植物密度与环境因子进行Pearson相关性分析,并根据相似度将11个属的浮游植物划分为4个类群(图6A):Group 1:长孢藻属,其与WT、DOC和氮营养盐呈正相关关系,与磷营养盐、Chl.a和APA呈负相关关系;Group 2:假鱼腥藻属、隐藻属、纤维藻属、拟柱孢藻属和栅藻属,它们与WT、DOC和氮营养盐呈负相关关系,与PO3-4-P、Chl.a和APA呈正相关关系;Group 3:转板藻属,其与WT和DN呈显著正相关关系;Group 4:直链藻属、小球藻属、平裂藻属和脆杆藻属,它们与环境因子的相关性较弱。通过PLS算法构建SEM,NFI=0.71,表明该模型对样本数据具有较好的拟合度[48](图6B)。在水体垂向混合期间,氮营养盐和DOC与WT呈正相关,而磷营养盐和浮游植物群落结构与WT呈负相关。水体Chl.a浓度与浮游植物群落结构呈正相关,但对氮营养盐和DOC浓度升高存在负响应。浮游植物群落结构与WT呈负相关,而与碳、氮、磷营养盐呈正相关,其中不同类群的浮游植物与浮游植物群落结构呈正相关。浮游植物群落组成的动态变化可以引起水体Chl.a浓度改变。
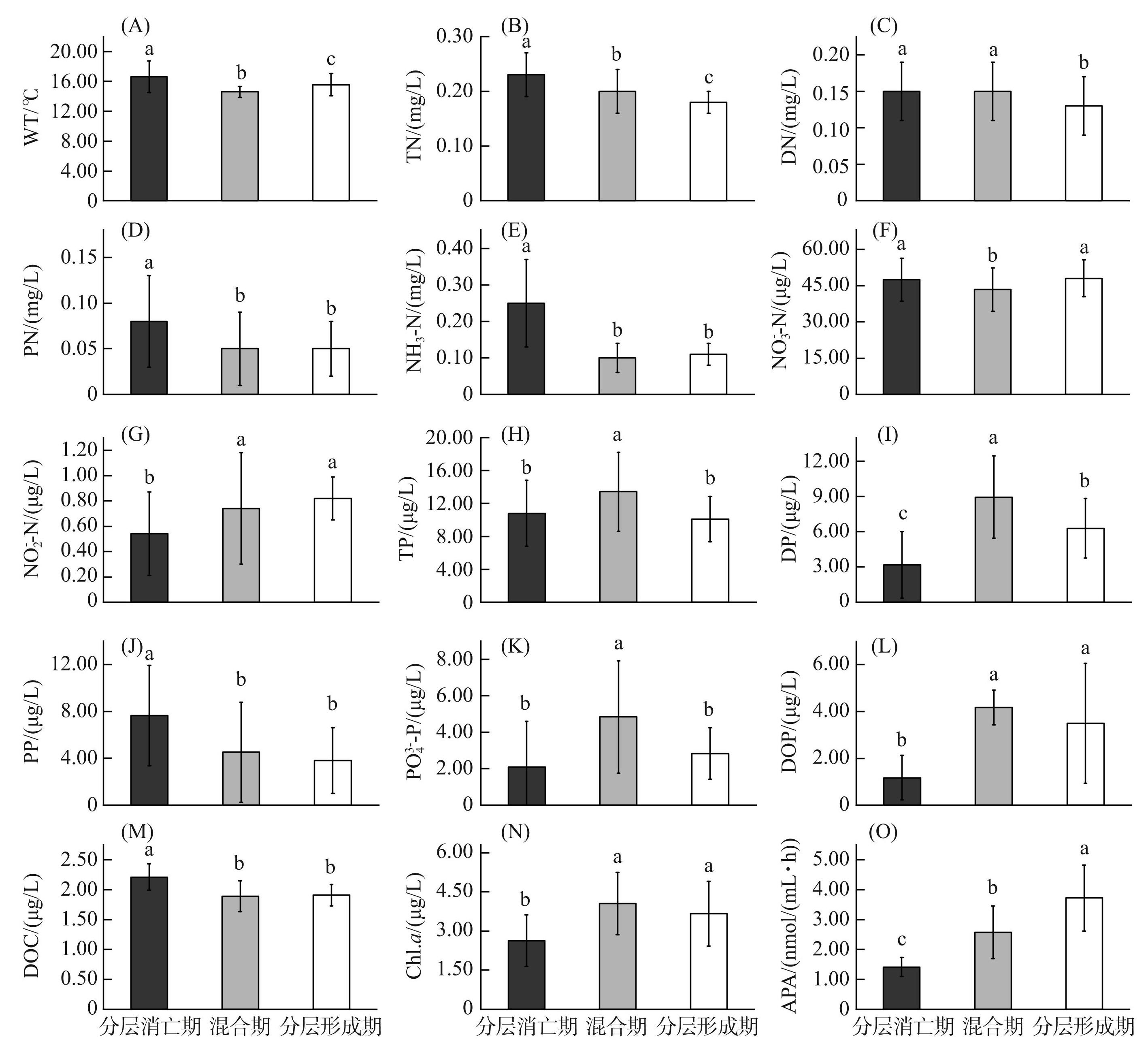
图2抚仙湖分层消亡期、混合期和分层形成期理化指标比较分析(图中展示的指标数据是0~60 m 水深范围内的平均值,不同小写字母表示组间具有显著性差异(P<0.05))
Fig.2Comparative analysis of physical and chemical indexes in the stratification decline period, mixing period and stratification formation period of Lake Fuxian (The indicator data in the figure are the average values across the 0-60 meter depth range, and different lowercase letters indicate significant differences among groups (P<0.05) )
3 讨论
3.1 深水湖泊水体混合过程对营养盐分布的影响
抚仙湖是亚热带高原深水湖泊,水体热分层每年都经历形成、稳定、消亡和混合的季节性单循环演化过程,对湖泊水环境的变化起着重要控制作用[6,49]。本研究观察到2022—2023年抚仙湖浮台位点0~60 m的垂向水温变化存在显著的季节特性(图1)。在2022年11月—2023年4月期间,抚仙湖水体经历了热分层失稳、混合、热分层重建的过程,在这个过程中,除了水温的变化外,表征营养盐的各因子也出现了同步的响应。与分层消亡期相比,混合期内大部分氮营养盐减少、磷营养盐增加(图2)。这与其他湖泊类似,如王吉毅等[49]在千岛湖也观察到在季节交替过程中水体关键环境指标存在明显的垂向变化,但春、秋两季氮和磷营养盐浓度的变化趋势相反。
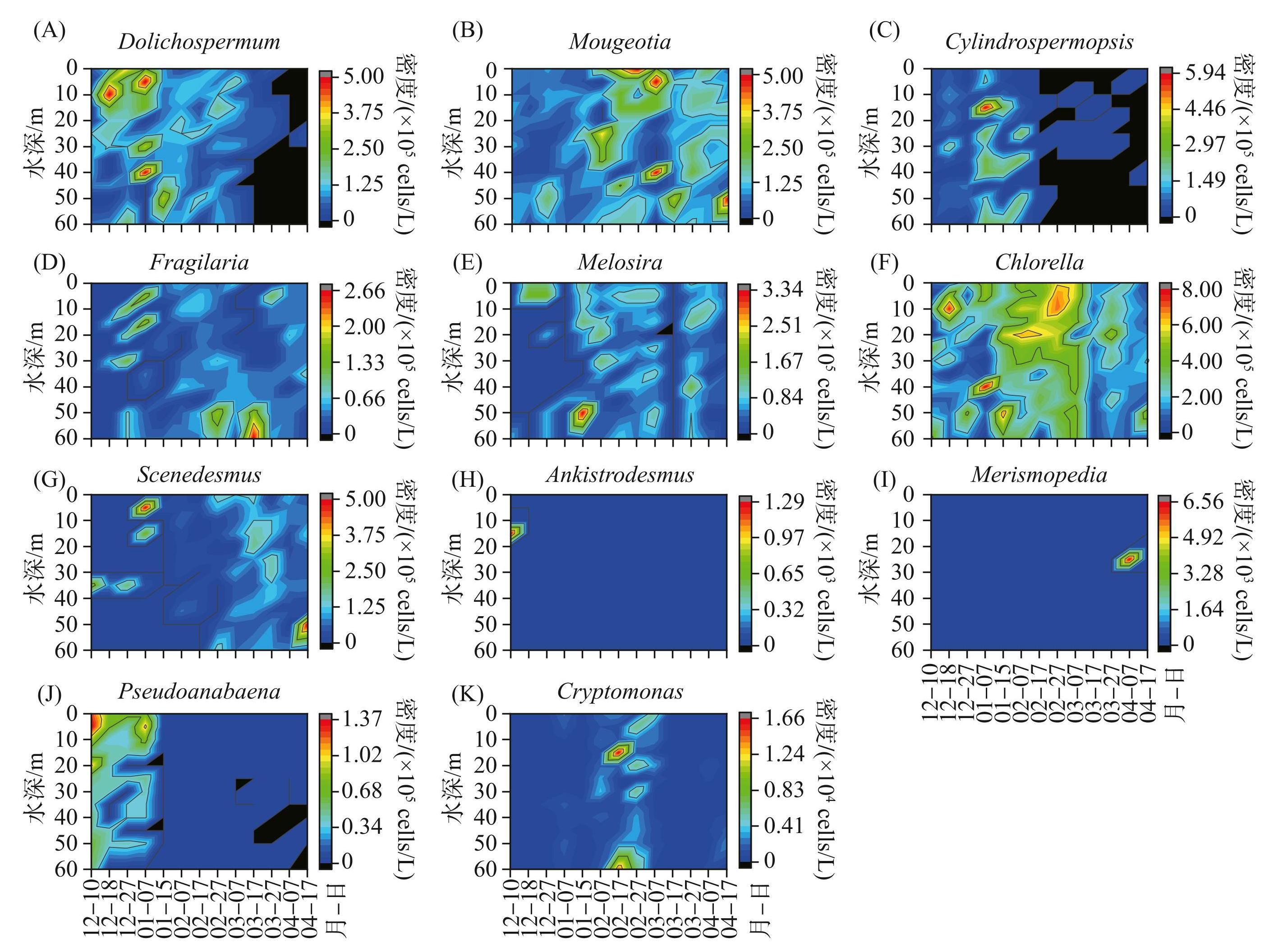
图3抚仙湖浮游植物密度时空变化特征:(A)长孢藻属;(B)转板藻属;(C)拟柱孢藻属;(D)脆杆藻属;(E)直链藻属;(F)小球藻属;(G)栅藻属;(H)纤维藻属;(I)平裂藻属;(J)假鱼腥藻属;(K)隐藻属
Fig.3Temporal and spatial variation characteristics of phytoplankton density in Lake Fuxian: (A) Dolichospermum; (B) Mougeotia; (C) Cylindrospermopsis; (D) Fragilaria; (E) Melosira; (F) Chlorella; (G) Scenedesmus; (H) Ankistrodesmus; (I) Merismopedia; (J) Pseudoanabaena; (K) Cryptomonas
冬、春季(11—4月)抚仙湖先后经历分层消亡、混合和形成的过程,期间引发水体垂向上强烈的对流混合,这不仅会造成湖体短期内突发性的物理变化,还可能扰动湖底沉积物—水界面,导致内源磷等营养盐迁移至上层水体中[19]。由于抚仙湖在2022年12月18日水体已完全混合,沉积物中释放的内源磷可能随着水动力过程迁移到上层水体中[50-51],导致混合初期上层水体中检测到较高的PO3-4-P浓度,但随着水体中浮游生物对PO3-4-P的利用,水体中PO3-4-P浓度会逐渐降低(从混合期到分层形成期经历了先升后降,图2K),故抚仙湖在混合初期的上层水体出现了短暂的PO3-4-P浓度增加现象。
3.2 水体混合对浮游植物群落动态变化的影响机制
在混合期抚仙湖大部分氮和磷营养盐分别处于消耗和增加的动态过程中(图2),而Chl.a浓度和APA显著增加;据此推测,在混合期抚仙湖浮游植物可直接利用的DIP浓度低[52-53],因此,混合期DOP在APA的作用下转化为浮游生物可直接利用的磷形态,而浮游植物密度随着磷浓度的增加而增加,并导致氮浓度不断降低。但同时期Chl.a浓度并未大幅升高(图2),这可能是因为冬、春季水温和光照强度等因素的影响,并未引起浮游植物密度的大幅升高[19-20]。由此可见,秋、冬季气温骤降导致湖泊水体垂向混合,对湖泊水环境产生重要影响,但其影响的深度和环境效应受到水温等因素的控制,因此在预测该类突发性水质变化事件及其对环境影响时应予以考虑。
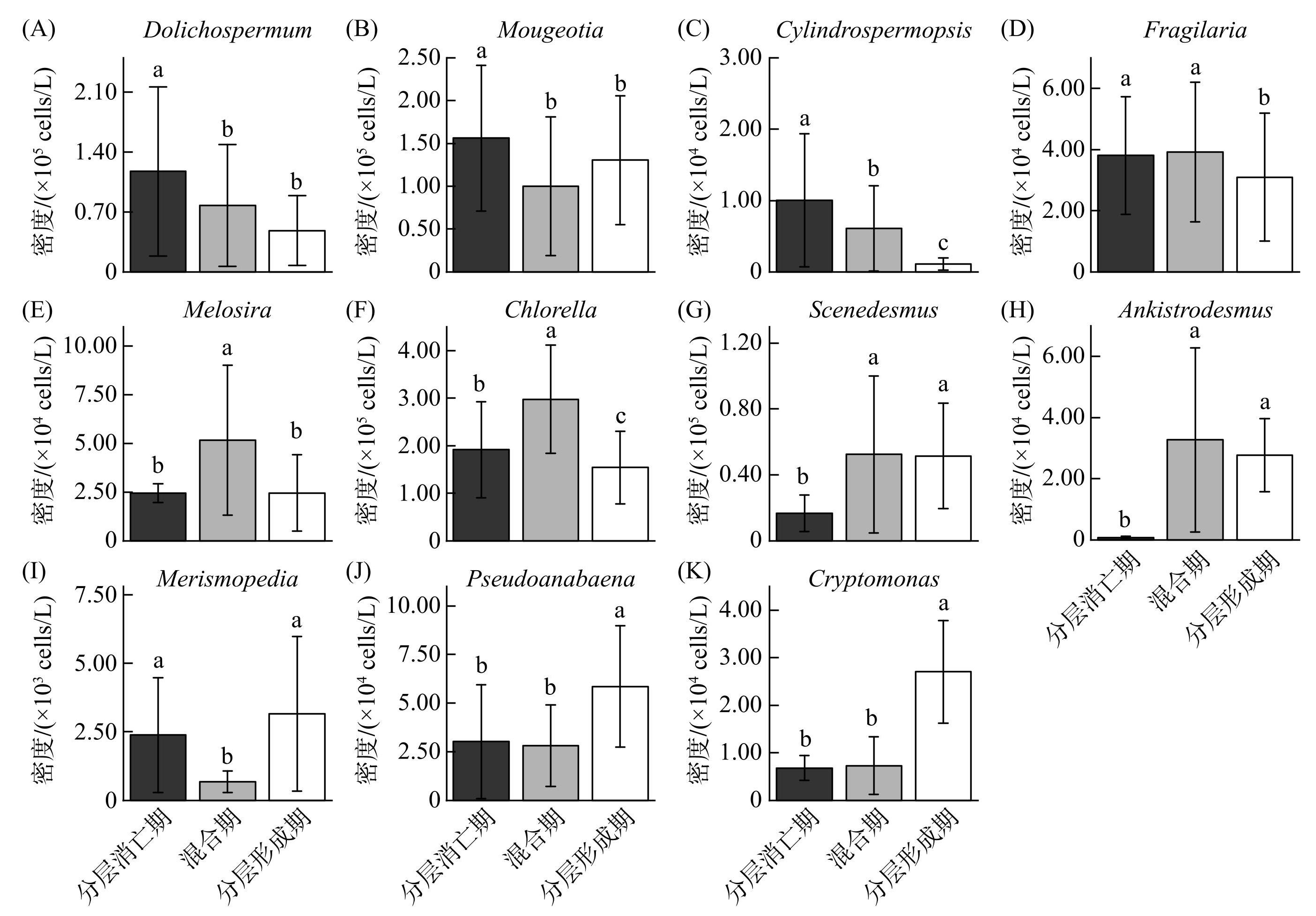
图4抚仙湖分层消亡期、混合期和分层形成期浮游植物密度比较分析:(A)长孢藻属;(B)转板藻属;(C)拟柱孢藻属;(D)脆杆藻属;(E)直链藻属;(F)小球藻属;(G)栅藻属;(H)纤维藻属;(I)平裂藻属;(J)假鱼腥藻属;(K)隐藻属(图中展示的浮游植物密度是 0~60 m水深范围内的平均值,不同小写字母表示组间具有显著性差异(P<0.05))
Fig.4Comparative analysis of phytoplankton density in the stratification decline period, mixing period and stratification formation period of Lake Fuxian: (A) Dolichospermum; (B) Mougeotia; (C) Cylindrospermopsis; (D) Fragilaria; (E) Melosira; (F) Chlorella; (G) Scenedesmus; (H) Ankistrodesmus; (I) Merismopedia; (J) Pseudoanabaena; (K) Cryptomonas) (The indicator data in the figure are the average values across the 0-60 meter depth range, and different lowercase letters indicate significant differences among groups (P<0.05) )
考虑到11个属的浮游植物对氮、磷营养盐变化的敏感度不同,本研究根据Pearson相关性一致性将11个属的浮游植物分为4个不同类群。其中长孢藻属和转板藻属分别与氮营养盐呈正相关、与磷呈负相关,而直链藻属、隐藻属、假鱼腥藻属、平裂藻属、拟柱孢藻属、栅藻属、纤维藻属、小球藻属和脆杆藻属则相反。这可能是因为抚仙湖中的浮游植物分别存在依赖氮营养盐和依赖磷营养盐的营养类型。此外,在分层消亡期长孢藻属和NH3-N浓度均有较高的峰值,混合期和分层形成期显著降低。对其他湖泊水库的研究中[49,54-55]也发现水温高的季节,氮浓度较高,主要以蓝藻门为优势;温度较低时以隐藻等为优势属。本研究中分层消亡期结束后水温下降,氮水平下降,长孢藻属的密度逐渐从峰值减退,隐藻等其他种类的浮游植物才相继达到较高密度。长孢藻属是固氮蓝藻的重要类群之一[56-57],能将大气中的氮气通过生物固氮作用合成生物可直接利用的氮形态,一般为NH3-N [51-52];据此推测,在分层消亡期抚仙湖中存在生物固氮作用导致NH3-N浓度上升,而随着混合期和分层形成期固氮作用减弱,NH3-N浓度随着生物利用消耗和氮形态转换而降低。当抚仙湖的环境条件适合生物固氮作用发生时,可能会导致水体氮浓度升高,从而导致浮游植物群落结构和生物量的改变;同样,在混合期,随着沉积物中磷的释放和向上迁移,那些对磷有较高需求的浮游植物物种生物量也可能增加。这些变化最终会引起浮游植物群落的结构和组成发生变动。
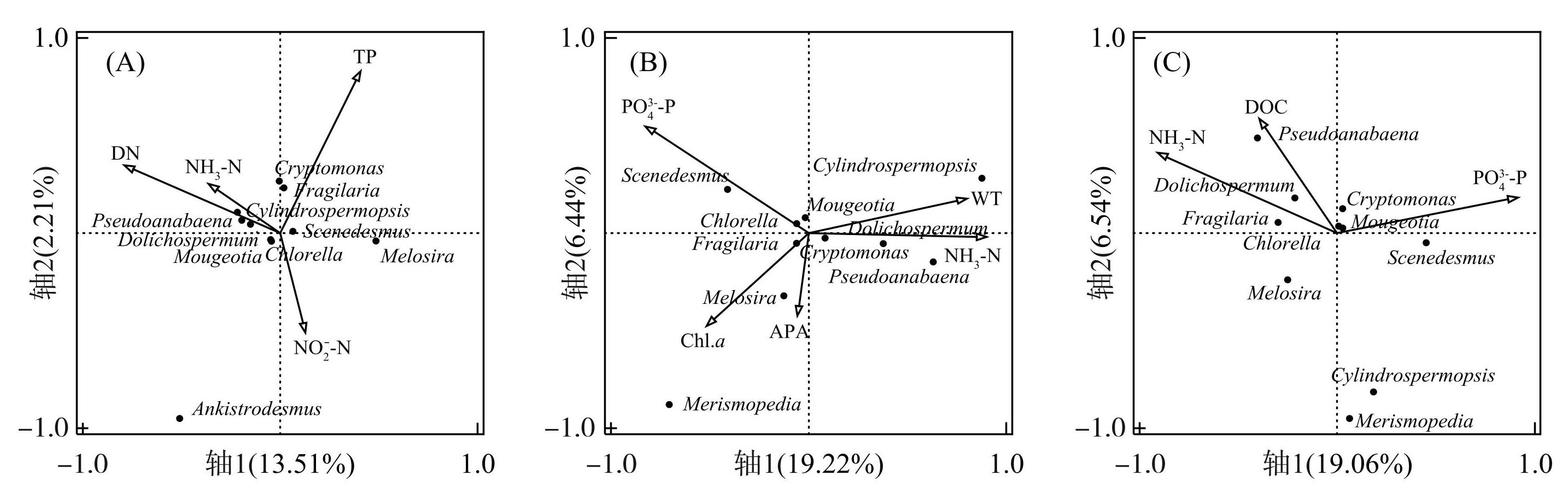
图5抚仙湖浮游植物与环境因子冗余分析:(A)分层消亡期;(B)混合期;(C)分层形成期
Fig.5Redundancy analysis of phytoplankton and environmental factors in Lake Fuxian: (A) stratification decline period; (B) mixing period; (C) stratification formation period
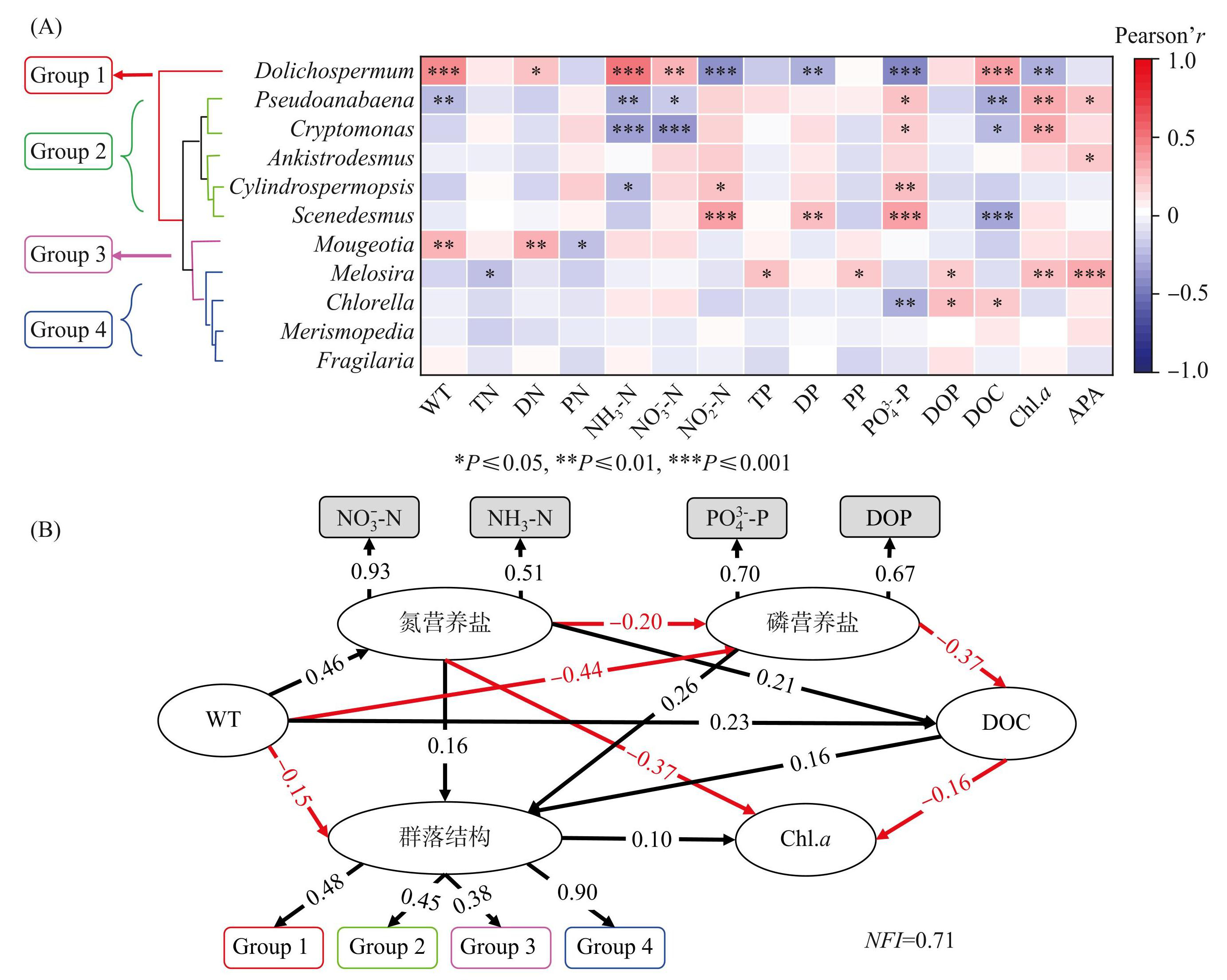
图6抚仙湖浮游植物密度与环境因子的Pearson相关关系(A)以及营养盐变化对浮游植物群落组成的影响机制分析(B)(图B中只展示显著相关(P<0.05)的线条,其中黑线表示正相关,红线表示负相关)
Fig.6Pearson correlation between phytoplankton density and environmental factors (A) , the mechanism of nutrient dynamics on phytoplankton community composition (B) of Lake Fuxian (In Fig.B, only significant correlations (P<0.05) are shown and marked by arrows in colors, where the black represents positive correlation and the red represents negative correlation)
进一步对混合期浮游植物的时空变化驱动机制进行分析发现,WT、NO-3-N、NH3-N、PO3-4-P、DOP和DOC等是驱动浮游植物群落变化的主要环境因子。而浮游植物群落时空变化的驱动机制主要表现在两个方面:一方面是受到不同营养盐的直接或间接驱动影响[58],另一方面是受到不同类别浮游植物的直接或间接驱动影响。在本研究中,浮游植物群落的变化主要受到营养盐浓度变化的影响;这种营养盐浓度的变化可能源于水温下降引起的沉积物中内源性磷的释放和迁移以及生物固氮作用的加强。这些因素共同作用,增加了水体中可供浮游植物利用的营养盐,从而影响了浮游植物群落的结构和动态。其中Group 1中长孢藻属可能通过固氮作用间接为其他物种提供氮源(可能通过共生、释放含氮化合物或者细胞死亡分解等形式),导致其他物种生物量变化[59]。在藻类竞争中,长孢藻生物量通常对水体氮水平较为敏感,而这种敏感性取决于水温[60];王吉毅等[49]在千岛湖也观察到水温和氮营养盐是驱动浮游植物优势属垂向分布的主要因素;本研究也同样观察到抚仙湖混合期内长孢藻与水温和NH3-N都呈显著正相关(图5B),而与分层形成期相比,混合期长孢藻的密度较高(105 cells/L,图3),对浮游植物总生物量贡献较大;因此,水温的作用可能有两个方面:一方面控制长孢藻密度变化,从而直接影响浮游植物生物量;另一方面可通过调节长孢藻密度控制NH3-N浓度水平,从而间接影响浮游植物总生物量。
本文发现在抚仙湖水体混合期间氮、磷营养盐是驱动抚仙湖浮游植物群落结构变化的主要环境因子,而有研究表明,抚仙湖底沉积物中磷的质量分数超过1%,是一个十分庞大的“磷库”[61-62],因此抚仙湖沉积物中内源磷的释放可能会导致湖水中营养盐浓度上升,甚至造成富营养化,导致湖水中溶解氧不足,从而对湖泊生态功能造成损害。此外,内源磷释放还会对水生植物和浮游动物的生态系统结构和功能产生影响,破坏湖泊的生态平衡[63]。因此为解决抚仙湖内源磷释放这一潜在问题,需要加强监测和保护。
4 结论
通过高频观测水体理化因子和主要浮游植物密度变化,本研究探索了深水湖泊抚仙湖水体垂直混合过程对浮游植物时空变化的影响过程和机制,主要结论如下:
1)在抚仙湖水体垂直混合期间,会引起含氮营养盐(特别是NH3-N)浓度显著降低,而含磷营养盐(PO3-4-P和DOP)浓度显著升高。
2)浮游植物11个属的时空分布特征不同,长孢藻属和拟柱孢藻属等蓝藻在分层消亡期密度高;而转板藻属和小球藻属在整个观测期密度均较高。水体混合期浮游植物群落结构变化率显著高于分层消亡期和分层形成期。
3)抚仙湖的水体垂向混合过程中,浮游植物群落结构时空变化主要受到WT、NO-3-N、NH3-N、PO3-4-P、DOP和DOC动态变化的影响。水体热力学分层变化改变营养盐分布特征和循环过程,影响深水湖泊初级生产过程。