摘要
随着气候变化和人类活动的增加,全球湖泊富营养化日趋加重,湖泊中蓝藻水华频繁发生。湖泊作为全球碳循环的热点区域,一方面通过浮游植物光合作用固定大气中的二氧化碳(CO2),另一方面又通过有机物的降解向大气中排放CO2和甲烷(CH4)。目前关于蓝藻水华对湖泊沉积物-上覆水系统中碳的源汇效应的研究主要集中于藻类死亡降解对含碳温室气体排放的影响,但对藻类光合作用固定大气中CO2效应的研究较少,因此蓝藻水华引起的湖泊碳的源汇效应尚不明确。本研究于2023年夏季采集太湖竺山湾的表层沉积物构建沉积物-上覆水系统,添加不同生物量的蓝藻,进行室内恒温水浴培养模拟实验,定期封闭培养柱,采集顶空气体样品,测定气体中CO2和CH4浓度及碳同位素丰度,研究蓝藻生长和衰亡对CO2和CH4产生途径及排放通量的影响。结果表明,蓝藻生长与衰亡阶段分别呈CO2吸收和排放状态,CO2排放通量随初始蓝藻添加量的增加而增加。蓝藻生长阶段CO2排放通量呈负值,藻类固定CO2;蓝藻衰亡阶段CO2排放通量随着蓝藻的死亡分解逐渐增加,在第25天达到稳定。蓝藻生长阶段的CH4排放通量为(0.022±0.011)~(3.159±0.51) μmol/(m2·h),在第7天达到峰值:蓝藻衰亡阶段的CH4排放通量显著升高,达到(0.219±0.017)~(9.783±0.215) μmol/(m2·h),并在第20天达到峰值。将CH4换算为CO2当量(CO2-eq),各处理组总的CO2-eq排放通量在蓝藻生长阶段表现为“碳汇”(-451.82~-113.28 mmol/(m2·h)),而在蓝藻衰亡阶段表现为“碳源”(35.46~196.86 mmol/(m2·h))。整个实验周期净CO2-eq排放通量为-746.71 mmol/(m2·h),表现出强烈的碳汇效应。13C-CO2和13C-CH4稳定同位素结果显示,表观分馏因子αC值均小于1.06,表明蓝藻生长和衰亡阶段CH4产生途径以乙酸发酵为主。随着初始蓝藻添加量的增加,αC逐渐减小,表明蓝藻提高了乙酸发酵途径在CH4代谢中的占比。研究结果对于揭示蓝藻水华对富营养化湖泊碳循环与源汇效应的影响机制具有重要科学意义。
Abstract
With climate change and intensive human activities, eutrophication of global lakes is increasing. Cyanobacteria blooms occur frequently in lakes. Lakes are the hot spots of global carbon cycle. On one hand, carbon dioxide (CO2) is fixed in the atmosphere through photosynthesis of phytoplankton. On the other hand, CO2 and methane (CH4) are emitted into the atmosphere through the degradation of organic matter. Current research on the effect of cyanobacterial bloom on carbon source and sink mainly focused on the effect of algae death and degradation on carbon-containing greenhouse gas emissions. There are few studies on the photosynthetic carbon sequestration effect of algae. Therefore, surface sediments effects of cyanobacterial blooms in lakes are not clear. In this study, surface sediments were collected from Zhushan Bay, Lake Taihu in summer of 2023 to form a sediment-overlying water system. By adding different biomass of cyanobacteria, indoor constant temperature water bath culture simulation experiments were conducted. The concentrations of CO2 and CH4 and the abundance of carbon isotope in the headspace were measured by sampling in the culture columns. Effects of cyanobacterial bloom's growth and decline on CO2 and CH4 production pathways and emission fluxes were studied. The results showed that cyanobacteria growth and decay correspond to CO2 absorption and emission states, respectively. The CO2 emission flux increased with adding of initial cyanobacterial. The CO2 emission flux was negative in the growth stage of cyanobacteria as algae fixed the CO2. CO2 emission flux during the decay stage increased gradually with the death and decomposition of cyanobacteria. It reached to stability on the 25th day. CH4 emission flux in the growth stage was (0.022±0.011)-(3.159±0.51) μmol/(m2·h) reaching its peak on the 7th day. CH4 emissions during the death stage increased significantly, reaching (0.219±0.017)-(9.783±0.215) μmol/(m2·h) with a peak on the 20th day. After converting CH4 to CO2 equivalent (CO2-eq) and summarizing, the total CO2-eq of each group showed as a “carbon sink” (-451.82--113.28 mmol/(m2·h)) during the whole experiment. However, it was a “carbon source” (35.46-196.86 mmol/(m2·h)) during the death stage. The net CO2-eq emission flux during the whole experiment period was -746.71 mmol/(m2·h), the water columns displayed a strong carbon sink effect. The results of 13C-CO2 and 13C-CH4 stable isotopes showed that the apparent fractionation factor αC were less than 1.06. It indicated that acetic acid fermentation was the main pathway of CH4 production in both growth and decay stages of cyanobacteria. αC gradually decreased with the increase of initial cyanobacteria supplement. This result indicated that cyanobacteria increased the proportion of acetic acid fermentation pathway in CH4 metabolism. These findings have important scientific significance in revealing the mechanism of cyanobacterial blooms on carbon cycling and source sink effects in eutrophic lakes.
Keywords
大气温室气体浓度升高导致的全球气候变暖给社会经济可持续发展以及人类生存带来巨大威胁,目前已成为备受关注的环境问题,其中二氧化碳(CO2)和甲烷(CH4)对气候变暖的贡献最为显著。湖泊作为内陆水圈的重要组成部分,是碳素生物地球化学循环的热点区域。湖泊每年接收大量的有机碳和无机碳,导致水环境中有机物负荷过高,表层沉积物中的有机碳随着时间的推移成为稳定的碳汇;另一方面湖泊生态系统中有机物的降解也会持续地向大气中排放CO2和CH4 [1]。尽管湖泊水体面积仅占全球陆域总面积的4%,但承接、储藏和输移的碳通量高达5.1×1015 g/a,占全球陆地年固碳通量的60%,并向大气排放的碳通量高达3.9×1015 g/a[2-3]。研究表明,太湖长年处于CO2饱和状态,CO2分压保持在(77.8±16.9)Pa,平均每年向大气排放(700±300)×106 t的碳[4-6]。然而,目前对于湖泊生态系统如何发挥碳汇成效以及碳汇价值如何估算仍未明晰。因此,研究湖泊生态系统的碳行为及其源汇效应对于全面认识全球碳循环、探究温室气体形成机制、构建更准确的温室气体预测模型均具有重要意义。
湖泊碳循环受到多种因素影响,藻类繁殖是其中的重要生物因素。藻类对湖泊碳循环具有双重效应,不仅能够通过光合作用固定CO2 [7];还会通过聚积、衰亡改变水体的理化性质,并释放易降解的有机质,为微生物的CO2和CH4代谢提供充足的底物。研究表明,在藻类大量繁殖的富营养化水体中,CO2和CH4的释放通量显著升高[8]。自然碳汇损失造成的温室气体排放加速了全球气候变暖,而气候变化对藻类生长的促进作用造成了自然碳汇的进一步损失,陷入温室气体排放的恶性循环。随着湖泊富营养化的发展,湖泊对于碳的源汇效应可能发生转换,并影响全球碳循环[9]。因此,在应对气候变化对策的选择上,需要兼顾多种温室气体净排放的减缓效应,既要充分发挥生态系统的固碳潜力,又要避免其他温室气体变化可能带来的气候和环境的负面效应,这是一个重大且极具挑战的前瞻性科学问题。
近几十年来,受人类活动和气候变化的双重影响,湖泊蓝藻水华频繁发生[10],导致其在区域碳循环中的稳定性大大降低,湖泊碳排放通量的量化和源汇转换的临界点是当前碳循环与环境变化关注的焦点。春季蓝藻在适宜的光照、温度及气候条件下,大量生长直至暴发形成肉眼可见的水华。随着时间推移,蓝藻进入衰亡期,在温度、光强、DO、营养盐水平降低的环境条件下,蓝藻因为细胞中糖类的积累和氧气泡的减少导致气囊破裂引起浮力下降,藻类沉降至表层底泥。蓝藻水华的暴发与衰亡影响着CO2和CH4的排放通量[11-14]。由于CH4在百年尺度上的单分子增温效应是CO2的30倍,CH4生成量的增多预计会对气候变化造成更严重的影响[15-17]。巢湖表层水体年平均CO2浓度为26.27 μmol/L,与Chl.a浓度呈显著负相关性,相关性随营养盐浓度的增加而增加[7]。悬浮在水体中的蓝藻衰亡后,残体分解并沉降至沉积物表面,为沉积物带来大量乙酸、甲基硫醚等易降解的有机质,这些有机质能促进沉积物中CH4的产生[18-21]。长期监测数据表明,富营养化湖泊中大量的蓝藻对CH4释放有促进作用[22-23],但均未阐明不同水华阶段(暴发和衰亡)对CO2、CH4释放的影响。根据产甲烷古菌的代谢途径,CH4的产生一般分为甲基营养型、乙酸营养型和氢(H2 /CO2)营养型3种类型[24],可以通过碳同位素特征进行区分。然而,蓝藻的生长与衰亡如何影响CO2和CH4之间的平衡尚不清楚。因此,探究蓝藻生长与衰亡的动态过程中,CO2和CH4排放通量的量化以及来源途径的确定对应对气候挑战、达成“双碳”目标具有十分重要的意义。
太湖是我国第三大淡水湖泊,是长江三角洲地区重要的水源地。作为浅水湖泊,外源营养盐输入与内源污染的双重压力导致太湖更容易出现富营养化。自2007年无锡饮用水危机事件以来,太湖的富营养化问题被高度重视并进行了高强度的治理,但至今蓝藻水华问题仍经年不断[25]。为探究不同强度的蓝藻水华对太湖CO2和CH4排放产生的影响,本研究于2023年8月在太湖竺山湾采集表层底泥和水华样本进行模拟培养实验,分析了不同生物量的蓝藻在生长和衰亡阶段对CO2和CH4生成途径和排放产生的影响。本研究有助于深入了解太湖蓝藻水华动态与CO2和CH4产排的关系,为有效控制CO2和CH4排放提供理论基础及科学指导。
1 材料与方法
1.1 采样点位及样品采集
于2023年8月在太湖竺山湾(31°28′10″N,120°06′27″E)进行沉积物样品采集(图1)。使用彼得森采泥器采集两箱表层5 cm的底泥,使用采水器和浮游植物网采集70 L湖水,使用YSI EXO2 7参数水质仪现场测定DO、pH和水温等理化指标。模拟实验开始前采集50 L新鲜湖水和500 mL浓缩藻液。样品采集后立即带回实验室处理。
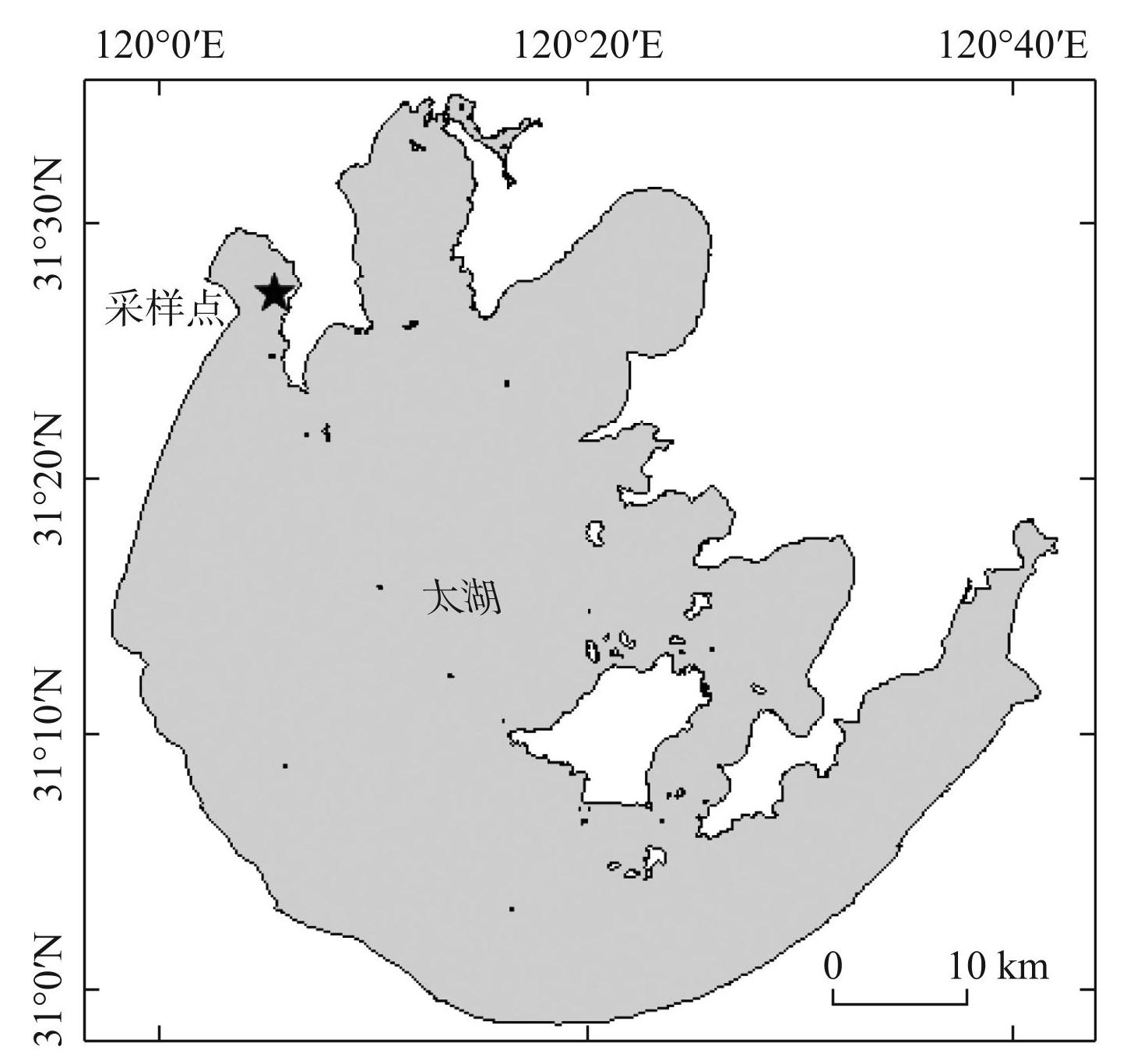
图1太湖采样点位设置
Fig.1Samping site setting in Lake Taihu
1.2 实验方案
将底泥混匀后重新静置进行为期1周的预培养,然后使用有机玻璃柱采集沉积物柱状样品,缓慢注入上覆水构成沉积物-上覆水系统,泥柱高度约为12 cm。使用浮游植物网(64 μm)过滤后借助蠕动泵注入有机玻璃柱至水面高度距顶部15 cm,此过程缓慢进行确保不扰动沉积物。另取3 根有机玻璃柱仅注入等体积的滤后湖水,底部隔开使水面高度与有底泥的培养柱相同。根据太湖的实际蓝藻水华情况,向培养柱的上覆水中加入浓缩藻液至Chl.a浓度分别为50、100和200 μg/L,模拟不同强度的蓝藻水华,加入NaNO3和KH2PO4使上覆水中的N、P浓度分别升高2和0.02 mg/L。
实验共设置5组不同的初始水华强度,分别记为N#空白组(仅湖水+底泥)、L#处理组(Chl.a浓度为50 μg/L湖水+底泥)、M#处理组(Chl.a浓度为100 μg/L湖水+底泥)、H#处理组(Chl.a浓度为200 μg/L湖水+底泥)、M-NS#处理组(Chl.a浓度为100 μg/L湖水+无底泥)(图2)。随机抽取3个泥柱进行破坏性取样,测定其底泥中的营养盐和碳浓度作为所有处理组底泥的初始值。设置水浴锅温度为采样当天实测温度(28℃),以8000 lx的光强进行12 h循环光照培养。
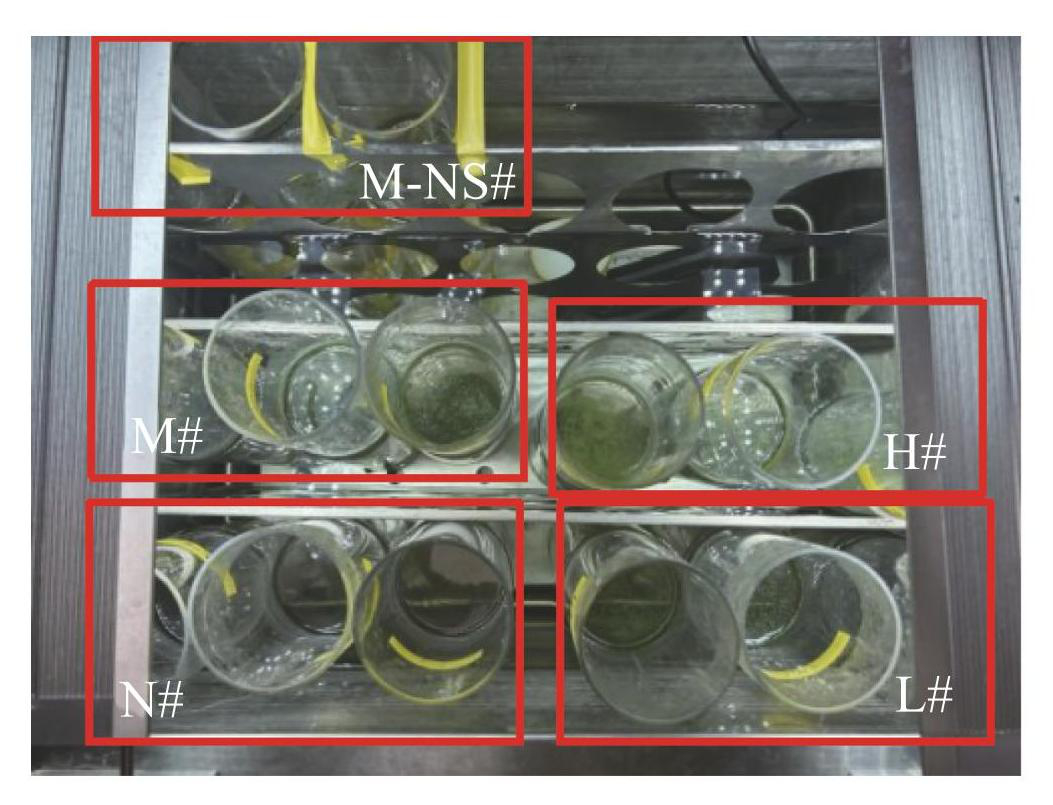
图2培养期间不同处理组差异
Fig.2Different treatment groups during culture period
实验持续30天,在培养的第1、4、7、10、15、20、25和30天进行取样并测定未扰动状态下上覆水的DO和pH,使用注射器采集上覆水用于测定其溶解性总氮(DTN)、溶解性总磷(DTP)、硝态氮(NO-3-N)、亚硝态氮(NO-2-N)、氨氮(NH3-N)。另取气样时,盖上合适的橡胶盖密封2 h,密封前取一次气体作为背景值,2 h后再取一次气体。取气时多次抽取、打回以混匀培养装置上部气体,然后通过注射器取出上部气体,注入预抽真空的气体样品袋中,待测。2 h后打开硅胶塞,采集沉积物上方5 cm处水样。取样和蒸发损失的水量使用过滤后的湖水进行补充。另在实验结束时进行破坏性取样,以测定总氮(TN)、总磷(TP)、Chl.a和表层2 cm沉积物的理化性质。
1.3 分析方法
1.3.1 水质分析
DTN、DTP浓度使用碱性过硫酸钾氧化、紫外分光光度法进行测定;NO-3-N、NO-2-N、NH3-N和磷酸盐(PO3-4-P)浓度使用Skalar 连续流动分析仪(荷兰Skalar公司,SAN++型)测定;Chl.a浓度使用热乙醇提取-分光光度法测定。CH4和CO2浓度使用Picarro G2201-i高精度碳同位素分析仪测定。
1.3.2 CH4和CO2排放通量测定
采用静态箱法进行CO2和CH4释放通量测定,具体操作流程为:将带有50 mL铝箔气袋的橡胶塞塞紧于模拟实验柱上端,并确保气密性。铝箔气袋主要是起到缓冲作用,避免因箱体内外压力差而被动漏气。用注射器抽取水体上方60 mL空气作为初始气体;关闭静态箱取气阀运行2 h后,用注射器通过取气阀缓慢抽取静态箱60 mL顶空气体,往复抽吸3次混匀后,回收60 mL气体作为运行结束后的气体。气体样品常温常压储存于300 mL气袋中。利用Picarro G2201-i高精度碳同位素分析仪完成测定。CO2、CH4释放通量按下式计算[26]:
(1)
式中,F为待测气体释放通量,mg/(m2·h); 为CH4或CO2浓度的变化率;ρ为标准状态下气体的摩尔密度,g/L;V为培养柱的顶空体积,m3;S为培养柱的面积,m2;T为室内的空气温度,℃。
参照第六次气候变化专门委员会政府间的评估报告(IPCC),核算CH4和整体的CO2当量(CO2-eq):
(2)
(3)
式中, 和 为CH4、CO2的CO2-eq,mmol/(m2·h);k1为一百年时间尺度下CH4的CO2-eq系数,为27.2; 为测量得到的CH4释放通量,mmol/(m2·h);FTotal为CH4和CO2的总CO2-eq。
1.3.3 CH4和CO2同位素计算
CO2和CH4之间的表观分馏因子(αC)计算公式如下[27]:
(4)
式中,αC为碳同位素分数,δ13C-CO2为CO2稳定碳同位素值,δ13C-CH4为CH4稳定碳同位素值。
1.3.4 数据分析
本研究中结果均为3组重复的平均值,误差棒为标准方差。数据预处理由Excel 2021软件进行,显著差异水平均采用P<0.05。使用SPSS 26软件对数据进行Shapiro-Wilk正态性检验和Levene方差齐性检验,对不符合方差齐性的数据进行对数转换,利用单因素方差分析的方法分析数据间的显著性差异,使用Origin 2018软件进行绘图。
2 结果与分析
2.1 上覆水DO和pH变化
实验期间,各处理组的水体DO浓度波动剧烈,在第0~10天升高,第10~20天下降,第20天后缓慢上升(图3a)。0~15天内,无蓝藻添加的N#组水体DO上升速度最快,第10天出现峰值(8.61±0.09) mg/L,其他处理组(L#~M-NS#)的DO浓度峰值分别为(6.7±0.91)、(7.87±0.11)、(7.08±0.79)和(7.98±0.63) mg/L。第16~30天各处理组的蓝藻衰亡加剧了水环境变化,DO均迅速下降,第20天出现最低值,分别为(2.59±0.59)、(3.56±0.14)、(3.57±0.22)、(2.86±0.29)和(4.00±0.49) mg/L(图3a),随后逐渐恢复。结果表明,随初始蓝藻添加量的增加,DO浓度变化越明显。
pH变化幅度较小,介于7.3~8.6之间,呈弱碱性。各处理组pH的变化趋势与DO浓度相似(图3b)。第0~7天内pH先升高随后下降,最高值分别为8.16±0.01、8.16±0.13、8.40±0.16、8.62±0.03和8.11±0.03;在第20天pH达到最低值后缓慢上升,各处理组pH最低值分别为8.10±0.10、8.12±0.09、7.47±0.05、7.4±0.07和7.34±0.02。
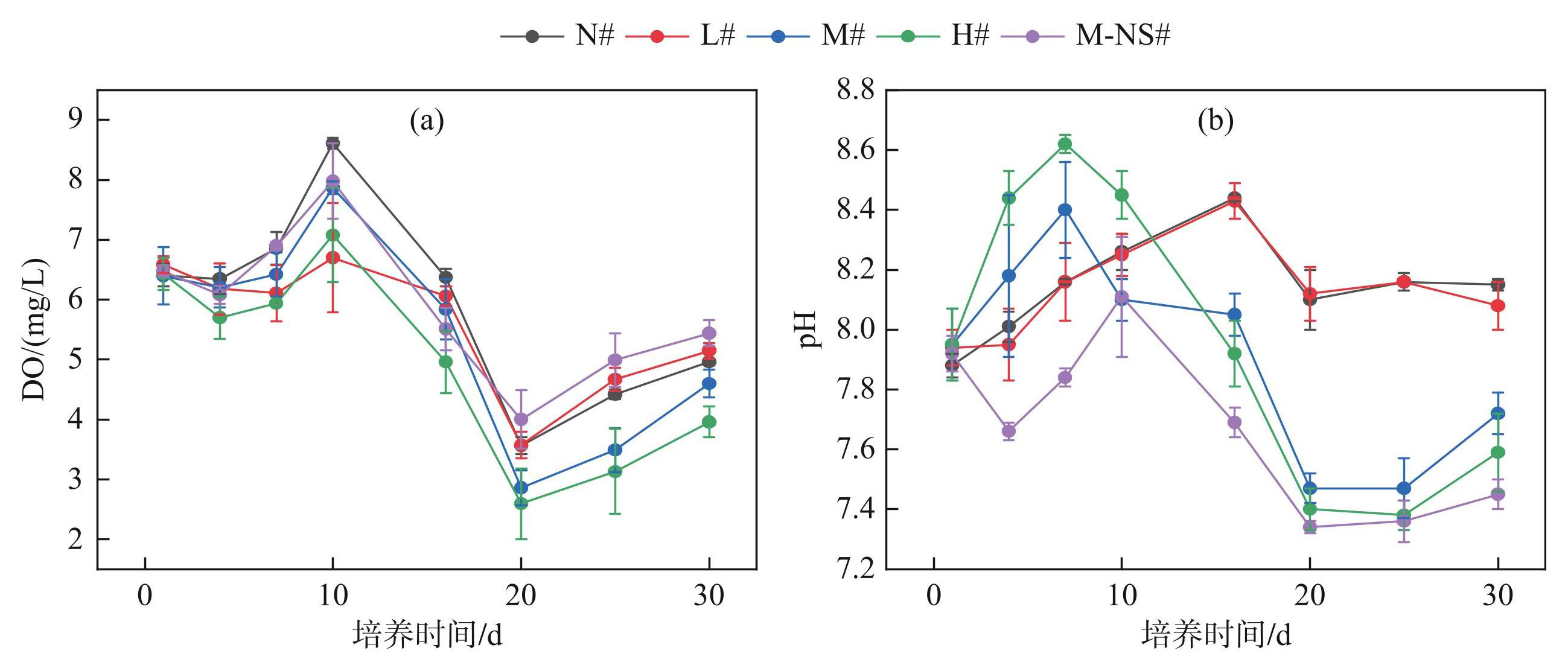
图3不同蓝藻添加量对上覆水DO(a)和pH(b)的影响
Fig.3Effects of different cyanobacteria additions on DO (a) and pH (b) in overlying water
2.2 上覆水氮磷浓度变化
随着底泥处理组的不同,各处理组上覆水的氮浓度存在明显差异(P<0.05)(图4)。有底泥处理组(N#~H#)的NO-3-N和DTN浓度在实验前期持续下降,下降速度随初始蓝藻添加量的增加而增快,并在第15天达到最低值,NO-3-N浓度分别为0、(0.12±0.09)、0和0 mg/L,DTN浓度分别为(0.42±0.01)、(0.60±0.01)、(0.64±0.08)和(0.69±0.08)mg/L。无底泥处理组(M-NS#)的NO-3-N和DTN浓度整体呈上升趋势,并在第10天出现峰值,分别为(2.66±0.23)和(3.54±0.29)mg/L(图4a和b)。在第15~30天,各处理组(N#~M-NS#)的NO-3-N和TN浓度缓慢升高,N#~H#组从第25天开始趋于稳定。
有底泥处理组(N#~H#)NH3-N和NO-2-N浓度在第0~15天内稳定在较低水平,无底泥处理组(M-NS#)浓度明显升高(图4c和d)。第15天后,各处理组的NH3-N和NO-2-N浓度均出现大幅波动,变化幅度随初始蓝藻添加量的增多而增大, NH3-N浓度在第20天出现峰值,分别为(0.35±0.03)、(0.15±0.09)、(0.44±0.08)、(0.52±0.05)和(0.23±0.01) mg/L。NO-2-N浓度在第25天出现峰值,分别为(0.26±0.05)、(0.17±0.08)、(0.37±0.20)、(0.41±0.23)和(0.73±0.57)mg/L。
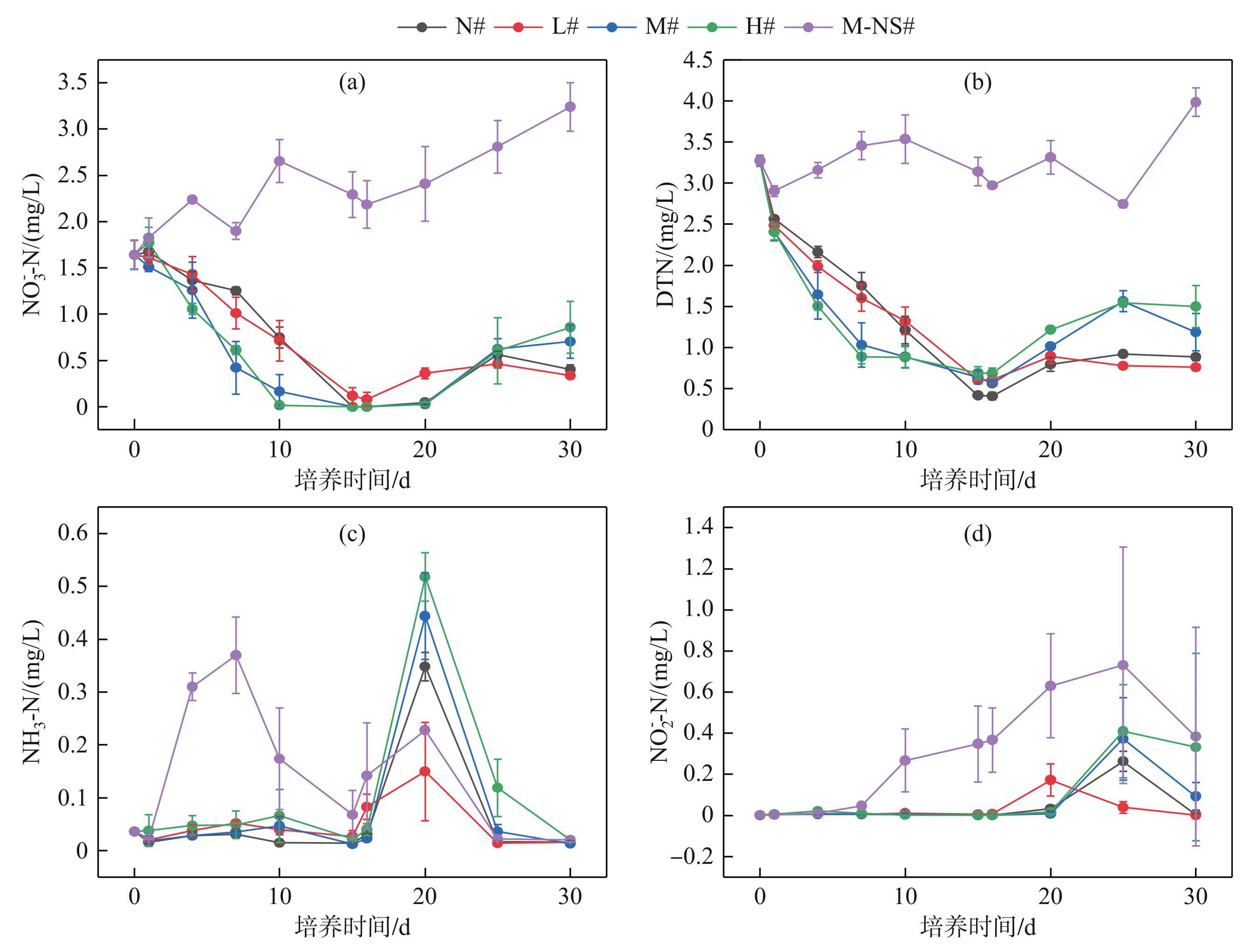
图4不同蓝藻添加量对上覆水氮浓度的影响
Fig.4Effects of different cyanobacteria additions on nitrogen concentration in overlying water
中、高蓝藻添加量处理组和无底泥处理组(M#、H#、M-NS#)的DTP和PO3-4-P浓度在第0~15天内呈波动下降,N#组和L#组PO3-4-P浓度在第7天出现峰值,分别为(0.056±0.004)和(0.049±0.005) mg/L,DTP浓度在第4~10天内维持在(0.077±0.01)~(0.089±0.01)mg/L之间。两个处理组的DTP和PO3-4-P浓度在第0~15天内均高于M#、H#、M-NS#处理组且波动幅度较大(图5)。
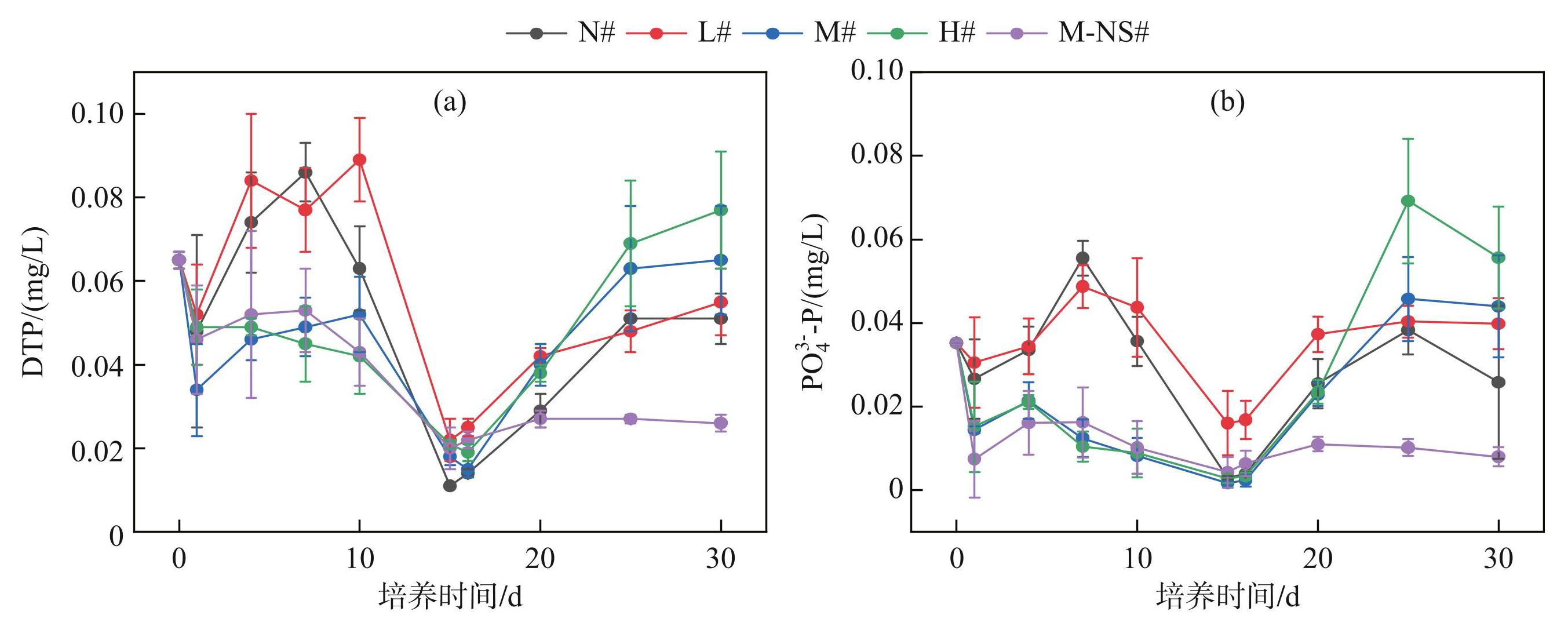
图5不同蓝藻添加量对上覆水磷浓度的影响
Fig.5Effects of different cyanobacteria additions on phosphorus concentration in overlying water
在第15~30天,有底泥处理组(N#~H#)DTP浓度逐渐上升,从第25天开始趋于稳定,并且随着初始蓝藻添加量的增加而不断升高。PO3-4-P浓度在第15~30天先升高后降低,峰值随初始蓝藻添加量的增加而增加。无底泥处理组(M-NS#)的DTP和PO3-4-P浓度在蓝藻分解后趋于平缓,无明显波动。
2.3 CO2和CH4排放通量变化
各处理组在不同培养时期CO2排放通量存在显著差异(P<0.05)(图6a)。第0~15天内各处理组的CO2排放通量呈负值,并且随着初始蓝藻添加量的增加而减小。无底泥处理组(M-NS#)的CO2排放通量波动范围最小。N#、L#和M-NS#组的CO2排放通量((-1.02±0.03)~(0.08±0.07) mmol/(m2·h))高于M#、H#组((-1.292±0.564)~(-0.528±0.116) mmol/(m2·h))。第15~30天内各处理组的CO2排放通量显著升高,并第15~16天内迅速上升,之后缓慢增加。N#、L#组的CO2排放通量介于(-0.303±0.013)~(-0.012±0.031) mmol/(m2·h)之间,M#、H#、M-NS#组的CO2排放通量介于(0.057±0.102)~(0.783±0.272)mmol/(m2·h)之间。
实验期间,各处理组的CH4排放通量分别在第7天和第20天各出现了一次峰值。各处理组(N#~M-NS#)的第1次峰值分别为(0.721±0.351)、(0.936±0.305)、(2.743±0.82)、(3.159±0.51)和(1.916±0.556) μmol/(m2·h);有底泥处理组(N#~H#)的第2次峰值分别为(4.268±0.155)、(2.687±1.52)、(9.783±0.215)和(4.462±0.714) μmol/(m2·h)。无底泥处理组(M-NS#)的CH4排放通量在第15~30天保持较低水平,无剧烈波动(图6b)。总体上,各处理组第0~15天的CH4排放通量显著低于第15~30天并随初始蓝藻添加量的增加而逐渐上升。
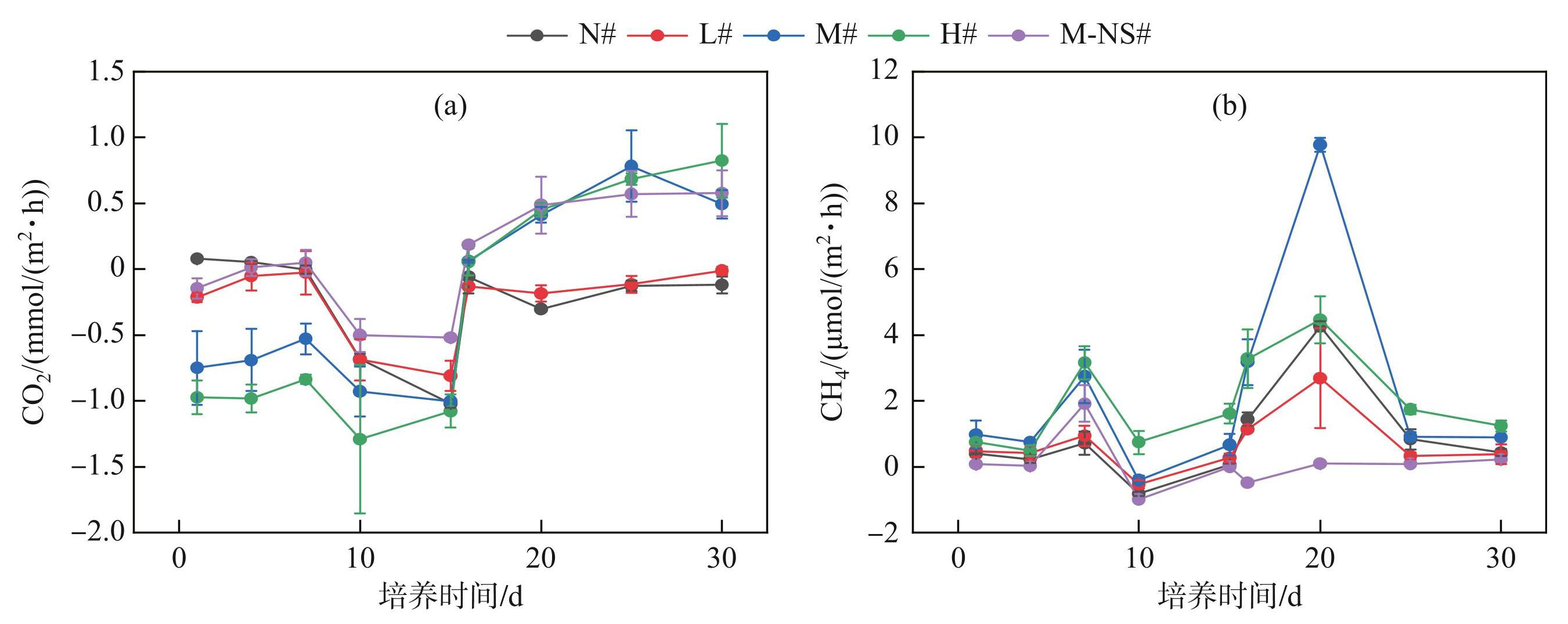
图6不同蓝藻添加量对沉积物CO2(a)和CH4(a)排放通量的影响
Fig.6Effects of different cyanobacteria additions on CO2(a) and CH4(b) emission fluxes in sediment
各处理组的总CO2-eq排放通量在第0~15天内为负值(图7a)。N#、L#和M-NS#组变化明显,总CO2-eq排放通量从(0.091±0.008) mmol/(m2·h)下降至(-1.019±0.032) mmol/(m2·h);M#、H#组波动较小,维持在(-1.272±0.555)~(-0.479±0.12) mmol/(m2·h)之间。第15天后总CO2-eq排放通量逐渐增加,N#和L#组的增幅较小,总CO2-eq排放通量保持负值,介于(-0.187±0.013)~(0.003±0.029) mmol/(m2·h)之间。M#、H#和M-NS#组的总CO2-eq排放通量上升迅速,从(-1.034±0.122) mmol/(m2·h)上升至(0.799±0.281) mmol/(m2·h),在第25天达到稳定。第0~15天各组的总CO2-eq排放通量维持在-4.189~-1.161 mmol/(m2·h)之间,第15~30天升至0.296~1.894 mmol/(m2·h)。第15天各处理组的总CO2-eq排放通量最低,达到-4.189 mmol/(m2·h),第25天最高,达到1.894 mmol/(m2·h)(图7b)。
不同蓝藻添加量处理组CO2-eq(CH4)排放通量在水华暴发期内随藻类生物量的增加而上升(图8a)。CO2-eq(CH4)排放通量从15.665 μmol/(m2·h)上升至183.289 μmol/(m2·h);在衰亡期,CO2-eq(CH4)排放通量与藻类添加量关系不明显(R2=0.284),随藻类添加量的增加波动加剧,在100 μg/L时达到最高,为401.45 μmol/(m2·h)。与CH4相反,CO2-eq(CO2)排放通量在水华暴发期随藻类生物量的增加逐渐下降(图8b),从-1.573 mmol/(m2·h)降到-5.163 mmol/(m2·h);到了蓝藻水华衰亡期,CO2-eq(CO2)排放通量与蓝藻添加量呈正比,但关系较为模糊(R2=0.792),排放通量最高值出现在200 μg/L时,达2.016 mmol/(m2·h)。总CO2-eq排放通量变化趋势与CO2一致(图8c),在水华暴发期,随蓝藻添加量的增多呈下降趋势,总CO2-eq排放通量在-5.008~-1.555 mmol/(m2·h)之间;水华衰亡期蓝藻添加量的增加提高了总CO2-eq排放通量,从0.417 mmol/(m2·h)提升至2.288 mmol/(m2·h)。
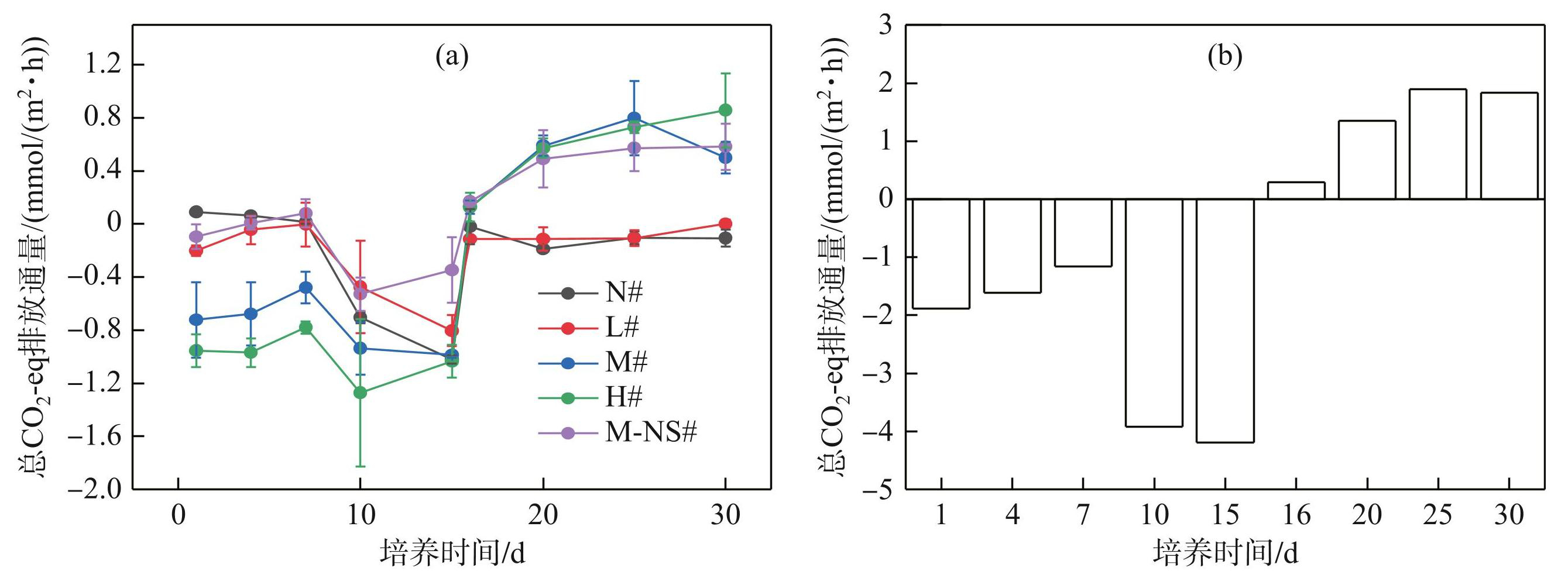
图7不同蓝藻添加量对沉积物总增温潜势的影响
Fig.7Effects of different cyanobacteria additions on the total warming potential in sediment
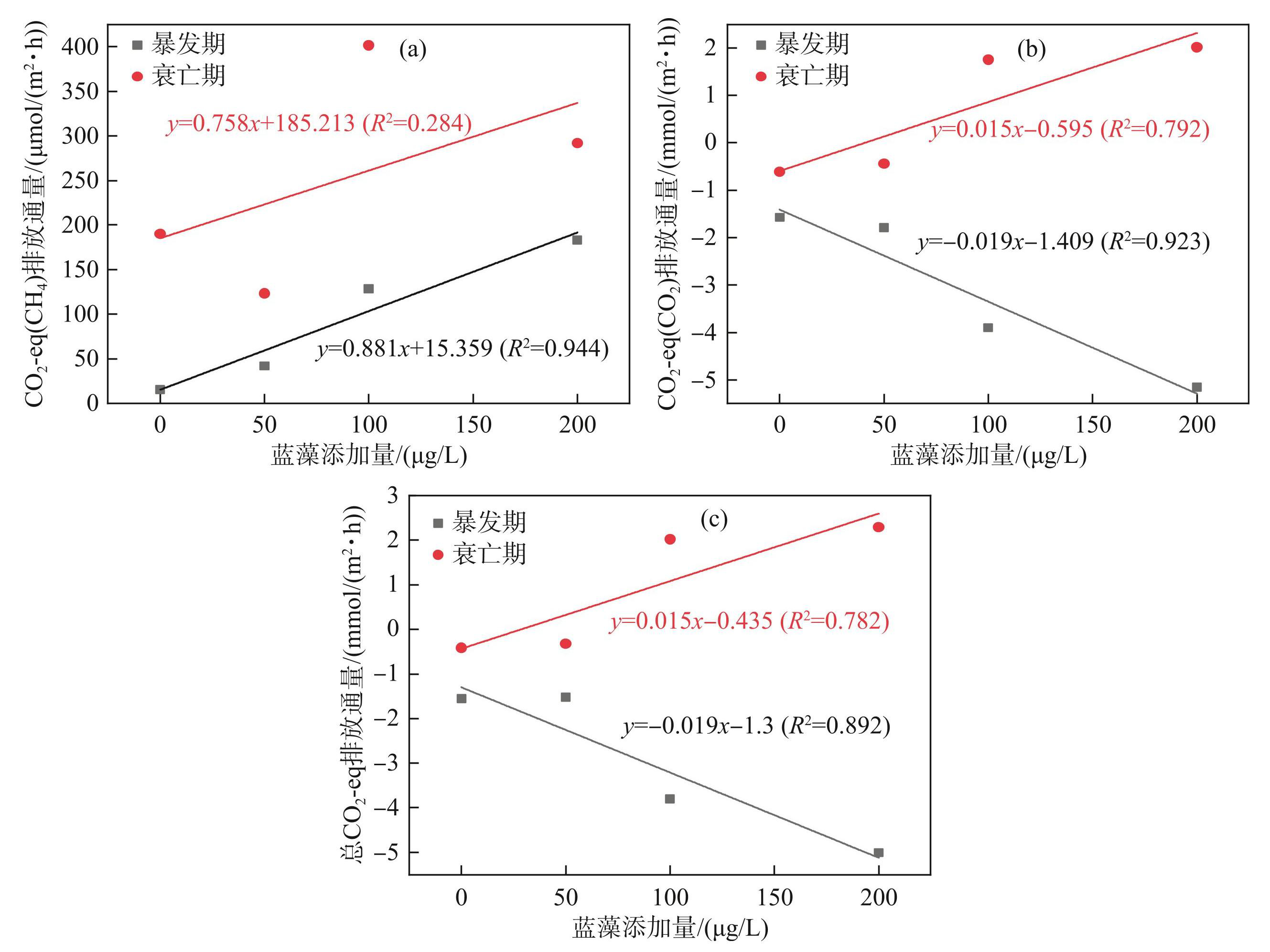
图8不同蓝藻添加量对沉积物CH4、CO2及总增温潜势的影响
Fig.8Effects of different cyanobacteria additions on CH4, CO2 and total warming potential in sediment
2.4 δ13C-CH4和δ13C-CO2稳定同位素变化
各处理组δ13C-CO2迅速降低,随后变化幅度放缓,至第25天后趋于平稳。各处理组第15~30天δ13C-CO2在(-14.71‰±0.13‰)~(-12.16‰±0.06‰)之间,δ13C-CO2随初始蓝藻添加量的增加迅速下降。无底泥处理组(M-NS#)的δ13C-CO2远大于中、高蓝藻添加量处理组(M#、H#),与空白组、低蓝藻添加量处理组(N#、L#)维持在同一水平(图9a)。δ13C-CH4变化趋势与CH4排放通量基本一致,培养期间分别在第7天和第25天出现2次峰值,第1次峰值出现后CH4排放通量迅速降低至最低点,第15天后又快速上升,第20天达到第2个峰值,随后再次下降并逐渐稳定在较低水平。无底泥处理组(M-NS#)在第15~30天内保持低水平的排放通量和δ13C-CH4(图9b)。除M-NS#组外,其他处理组δ13C-CH4的上升幅度随初始蓝藻添加量的增加而增大。各处理组(N#~M-NS#) δ13C-CH4的第1次峰值分别为(-47.79‰±1.49‰)、(-47.59‰±1.55‰)、(-47.60‰±0.82‰)、(-45.74‰±3.41‰)和(-42.89‰±1.61‰),底泥处理组(N#~H#)δ13C-CH4的第2次峰值分别为(-46.66‰±0.67‰)、(-47.84‰±1.45‰)、(-47.02‰±1.26‰)和(-46.22‰±0.20‰)。
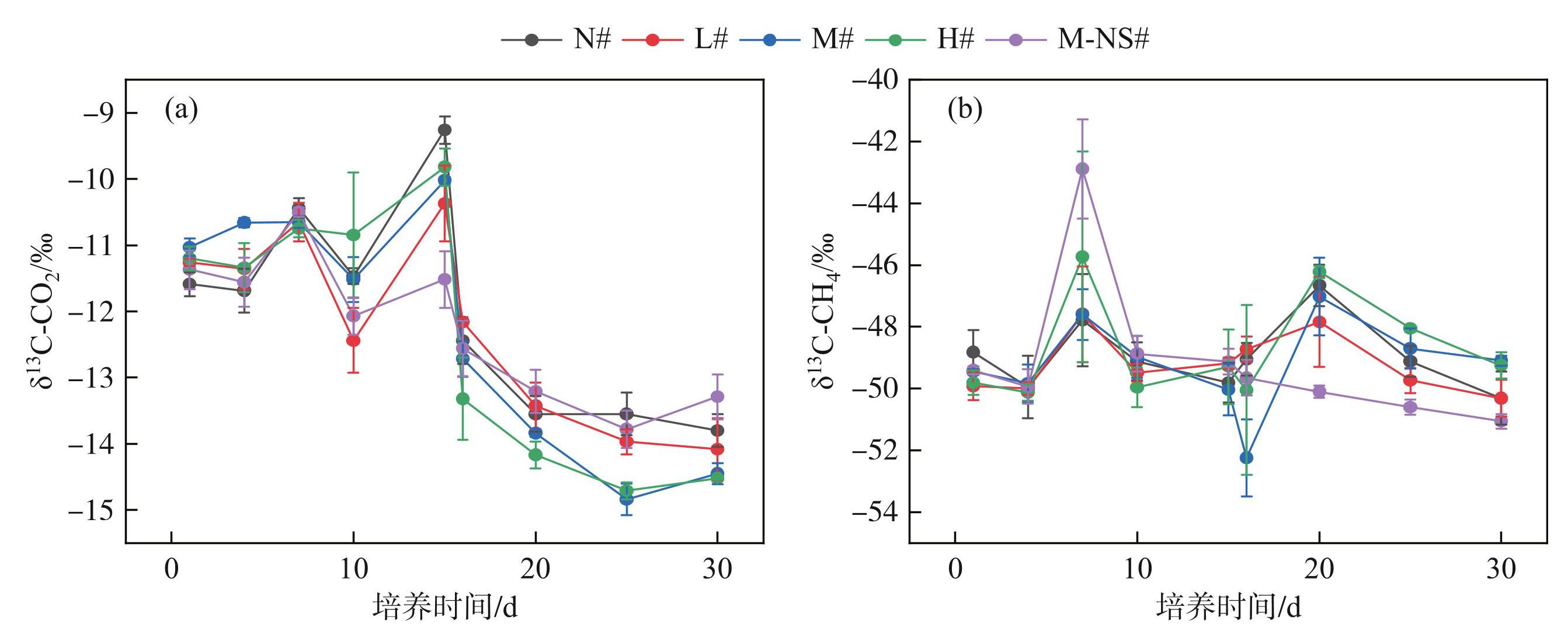
图9不同蓝藻添加量对沉积物δ13C-CO2和δ13C-CH4的影响
Fig.9Effects of different cyanobacteria additions on δ13C-CO2 and δ13C-CH4 in sediment
2.5 CO2、CH4表观分馏因子变化
实验期间,各处理组(N#~M-NS#)的αC均小于1.06,变化范围分别为1.035~1.043、1.036~1.041、1.035~1.042、1.034~1.042和1.034~1.040(图10)。第0~15天内,所有处理组的αC先下降,在第7天出现最低值后迅速上升,并在第15天达到最大值。第15~30天内,所有处理组的αC先下降,在第20天出现最小值后缓慢上升。随着初始蓝藻添加量的增加,αC逐渐降低。无底泥处理组(M-NS#)的αC在第0~15天最低,在第15~30天最高且无明显波动。
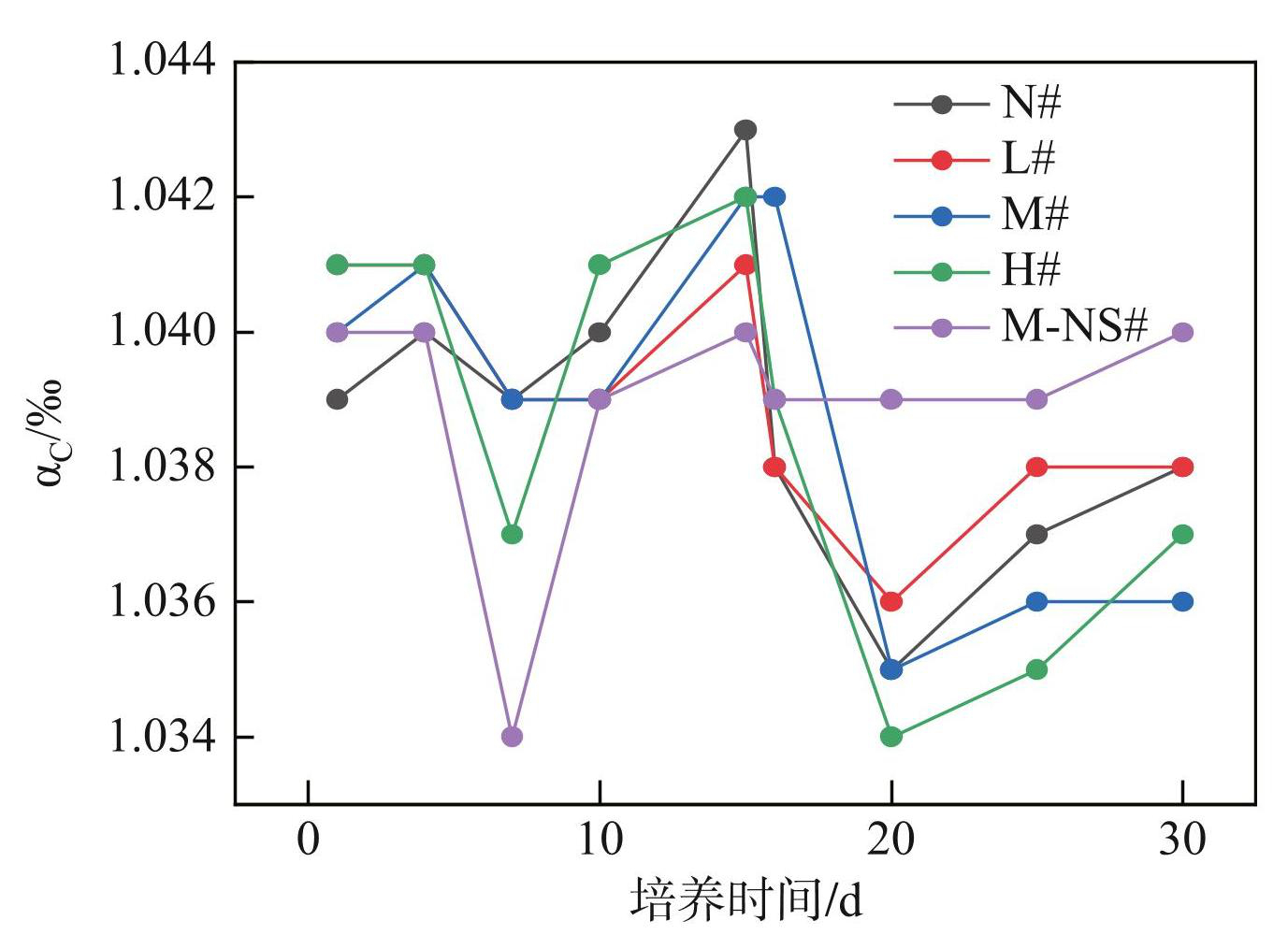
图10不同蓝藻添加量对沉积物CO2和 CH4表观分馏因子的影响
Fig.10Effects of different cyanobacteria additions on apparent fractionation factor of CO2 and CH4 in sediment
3 讨论
3.1 蓝藻生长与衰亡对CO2、CH4排放通量和增温潜势的影响
实验期间,有底泥组(N#~H#)的NO-3-N、DTN浓度在第0~15天内持续下降,第15天基本耗尽,随后缓慢升高,pH在第15天后迅速下降,表明蓝藻在培养前15天处于正常生长状态(生长阶段),在营养盐逐步耗尽之后大量衰亡降解(衰亡阶段)(图4和图5)。
蓝藻生长阶段,强烈的光合作用吸收CO2,使CO2排放通量呈负值(图6a),与Zhang等[28]在巢湖西北湾水华时期的野外监测结果一致。无底泥组(M-NS#)的CO2吸收较弱,可能是由于没有底泥的内源供给导致蓝藻生长状态不佳,光合作用减弱,限制了对CO2的吸收。唐千等[29]的研究发现,沉积物和藻类共存条件下的Chl.a浓度明显高于无沉积物的处理。生长阶段过后,蓝藻的衰亡降解迅速降低了DO水平,释放出的有机质为CH4的产生提供了充足的底物,CH4厌氧氧化产生了大量的CO2 [22,30-33],使该阶段的CO2排放通量由负变正。
由于系统中CH4产生与消耗的动态平衡,有底泥组(N#~H#)的CH4排放通量在蓝藻生长(第7天)和衰亡(第20天)阶段各出现了一次峰值(图6b)。蓝藻衰亡阶段的CH4排放通量为生长阶段的1.4~6.3倍。蓝藻在生长期间通过光合作用改变水体的DO和pH,影响了微生物的产CH4活性,改变了好氧水体CH4的产排[18,34-38]。研究发现,参与产甲烷菌代谢的若干辅因子和酶(如CO脱氢酶和乙酰辅酶a合成酶)受氧气的影响显著,当DO浓度高于10.0 mg/L,产甲烷菌活性将被完全抑制[39],DO通过影响CH4的氧化速率来调节CH4浓度。Maruya等[40]在Abashiri湖的研究表明,当DO浓度介于0~3.0 mg/L时,CH4通量恒定在1.4 mmol/(m2·d);当DO浓度介于3.0~6.0 mg/L时,CH4通量迅速下降到0.4 mmol/(m2·d)。除DO外,产甲烷菌的活性还受到水体pH的影响,产甲烷菌对pH值的变化非常敏感,通常在中性或弱碱性环境条件下最为理想[26]。本实验开始的第1~7天内,培养柱上覆水的pH升高,DO未出现明显升高,产甲烷菌在弱碱性水体中并未受到DO抑制,活性增强,CH4排放通量增加;第7~10天内,培养柱上覆水的pH下降,DO迅速升高,产甲烷菌活性受到抑制,CH4排放通量减少。蓝藻生长阶段吸收大量的CO2作为碳营养源储存在细胞中,随后在蓝藻衰亡阶段释放易降解的有机质,被产甲烷菌利用并快速转化为CH4和CO2[41-42]。在第15~20天,CH4的释放通量快速升高,并在第20天后随着有机质的减少而受到限制。此外,氧化还原条件也调控着有机碳的分解方式。相比于氧化条件,还原条件更有利于有机碳向产CH4的方向分解[31-32],同时抑制CH4的氧化消耗,从而造成CH4的大量释放[33]。研究发现,富营养化湖泊藻类聚集区存在大量的CH4积累与释放[43]。无底泥组(M-NS#)没有底泥内源供给的调节,蓝藻生长缓慢且消亡速度快,造成生长阶段和衰亡阶段CH4的低水平排放(图6a)。张楠等[44]的研究发现,蓝藻降解过程中水体DO以及沉积物间隙水中的SO2-4浓度降低,有利于CH4的产生,同时促进了下层沉积物的CH4产生过程。外界学者多集中于研究蓝藻衰亡对湖泊碳循环的影响,对蓝藻全生命周期影响湖泊碳循环的研究较少。本研究表明水华暴发时蓝藻对水体理化性质的改变作用和对大气中CO2的固定作用对湖泊碳循环有深远影响。
实验周期内,CH4的CO2-eq排放通量远小于CO2的CO2-eq排放通量,表明在蓝藻水华的暴发期和衰亡期CH4的增温潜势要小于CO2,尤其是蓝藻衰亡期。虽然CH4的增温效应远远大于CO2,但结果表明CO2高排放通量导致的CO2高增温潜势,直接影响湖泊蓝藻生长与衰亡对湖泊碳源汇效应的结果。不同蓝藻添加量处理组总CO2-eq排放通量在蓝藻生长阶段在-4.189~-1.161 mmol/(m2·h)之间,而在蓝藻衰亡阶段则升高至0.296~1.894 mmol/(m2·h),实验过程中总CO2-eq排放通量为-7.385 mmol/(m2·h)(图7),表明蓝藻的生长会使湖泊成为碳汇,而蓝藻的衰亡则会将湖泊转变为碳源。随着初始蓝藻添加量的增加,水体在蓝藻生长阶段对CO2的吸收作用和在蓝藻衰亡阶段的排放作用均不断增强,但整体表现为CO2的汇。以往的研究中,对于太湖作为碳源、碳汇单元的结论尚无定论[4,45]。本研究的结果表明,蓝藻的生长衰亡是湖泊作为碳汇单元的重要组成部分。
3.2 蓝藻生长衰亡对CO2、CH4来源途径的影响
CH4产生的主要生物途径是基于简单有机化合物的厌氧发酵,通过乙酸或甲基营养化产生[46],还可以在缺氧环境下利用H2消耗CO2产生[47]。此外,CH4氧化为CO2是减少CH4净排放的主要途径。由于CO2和CH4的同位素特征不同,可以通过δ13C-CH4、αC值和εC(表观分馏因子)值评估CH4的产生途径[27,48]。αC可以定性表征CH4产生过程的代谢途径,当αC大于1.06时,主要是CO2还原途径(氢营养途径),当αC小于1.06时,主要是乙酸发酵途径[49]。由于淡水湖泊中甲基化合物含量低,乙酸型和氢营养型是湖泊生态系统主要的CH4产生类型[24]。Hornibrook等[50]研究了各种淡水、海洋和陆地环境中αC随沉积物深度的变化,发现了两种不同的剖面类型,一种是αC随深度增加,表明依赖CO2的产CH4作用(许多淡水环境)越来越重要;另一种是αC在较高值下保持相对稳定,表明产CH4作用受限于CO2还原途径(海洋环境)。
实验期间,5个处理组的αC均小于1.06,表明蓝藻生长与衰亡过程的CH4产生途径主要以乙酸发酵为主。各处理组的αC均存在波动变化(图10)。其中,蓝藻生长和衰亡阶段的αC分别于第7天和第20天出现最低值,随后迅速上升,表明此时乙酸发酵途径的比例相对增加,造成CH4排放通量的突然增加。各处理组蓝藻衰亡期乙酸发酵途径所占比例均大于蓝藻生长期,造成CH4排放通量的同步变化。刘喜乐[51]基于δ13C-CH4、δ13D-CH4以及δ13C-CO2的研究表明,混合底物发酵体系产生的CH4稳定同位素更低,且不同底物体系均以乙酸代谢途径为主。张楠等[44,52]的研究发现蓝藻降解产物的增加会提高产甲烷菌丰度,导致蓝藻衰亡期CH4排放通量的增加。蓝藻生长阶段M-NS#组的αC最低,乙酸发酵途径占比最高,而蓝藻衰亡阶段M-NS#组的αC最高,乙酸发酵途径占比最低,表明底泥的存在影响了不同水华时期的CH4来源。随着初始蓝藻添加量的增加,δ13C-CH4和δ13C-CO2在蓝藻生长期升高,但在蓝藻衰亡期降低(图9),而αC在蓝藻生长和衰亡阶段均降低,表明高强度的蓝藻水华为产甲烷菌提供了更多的代谢底物,提高了产甲烷菌的丰度,有助于增加乙酸发酵途径在CH4来源中的占比[34]。
3.3 对蓝藻水华管理的启示
蓝藻水华改变了水体的DO和pH[15-17,53],并提供了充足的有机质,促进了CH4和CO2的产生。水华强度越高,DO和pH的波动越大,衰亡期输入的有机质更多,更有利于水环境中产甲烷菌的代谢。藻类降解不仅释放了氮、磷等营养物质[30],还产生了大量的有机碳,为温室气体的产生提供了易降解的底物,促进了温室气体的产生与排放[54]。本研究中,中、高蓝藻添加量组和无底泥组(M#、H#和M-NS#)的CH4和CO2排放通量均大于空白组和低蓝藻添加组(N#、L#)(图6)。蓝藻的不同阶段也影响了CH4和CO2的产生方式[22,30,42]。蓝藻生长阶段,初始蓝藻添加量的增加导致CH4排放通量提高了3.7~4.6倍,CO2排放通量相对减少[39-40];进入衰亡阶段之后,初始蓝藻添加量的增加提供了更多的有机质,促进产甲烷菌产生大量的CH4和CO2,导致CO2排放通量提高了6.2倍[31-33]。本研究中Chl.a浓度为0和50 μg/L的处理组CO2和CH4的排放通量接近并保持在低水平。随着Chl.a浓度的升高,处理组CO2和CH4的排放通量出现较高的增长,远高于Chl.a浓度为0和50 μg/L的处理组。随Chl.a浓度的持续升高,CO2和CH4排放通量大幅增加。因此,将湖泊水华强度控制在Chl.a浓度为50 μg/L以下至关重要,不仅可以限制CO2和CH4的排放,还能保持湖泊的碳汇效应。
目前国内外针对富营养化湖泊蓝藻水华问题多采取原位覆盖[54]、底泥疏浚[55]、水生植物恢复[56]和蓝藻打捞[57]等多项技术。其中,蓝藻打捞是一种简单易行、见效快、无二次污染的物理移除技术,已广泛应用于蓝藻水华的应急管理。打捞可以有效削减藻源污染物。截至2020年底,太湖蓝藻日打捞量已超过6×104 t。本研究结果表明,蓝藻的移除不仅可以减少氮、磷的释放,还能有效削减温室气体的排放,表现出减污和降碳的协同潜力。
4 结论
1)蓝藻生长与衰亡阶段对水体CH4和CO2排放的影响不同。在生长阶段,蓝藻通过光合作用吸收CO2,改变了水体DO和pH,对CH4和CO2排放通量产生影响,水体表现为对碳的吸收作用。在衰亡阶段,蓝藻增加了水体有机质并减少了DO,促进CH4和CO2的排放,水体表现为对碳的释放作用。
2)实验期间,αC值均小于1.06,表明所有处理组的CH4排放通量主要来源于乙酸发酵。蓝藻生长阶段的αC较大,乙酸发酵产生CH4的作用相对较小;蓝藻衰亡阶段的αC逐渐减小,乙酸发酵产生CH4的贡献增大。
3)不同蓝藻生物量对CH4和CO2排放通量具有显著的影响。初始蓝藻添加量越大,CH4和CO2的排放通量越大。因此应及时打捞蓝藻,避免蓝藻大量堆积,这有助于降低温室气体的排放。
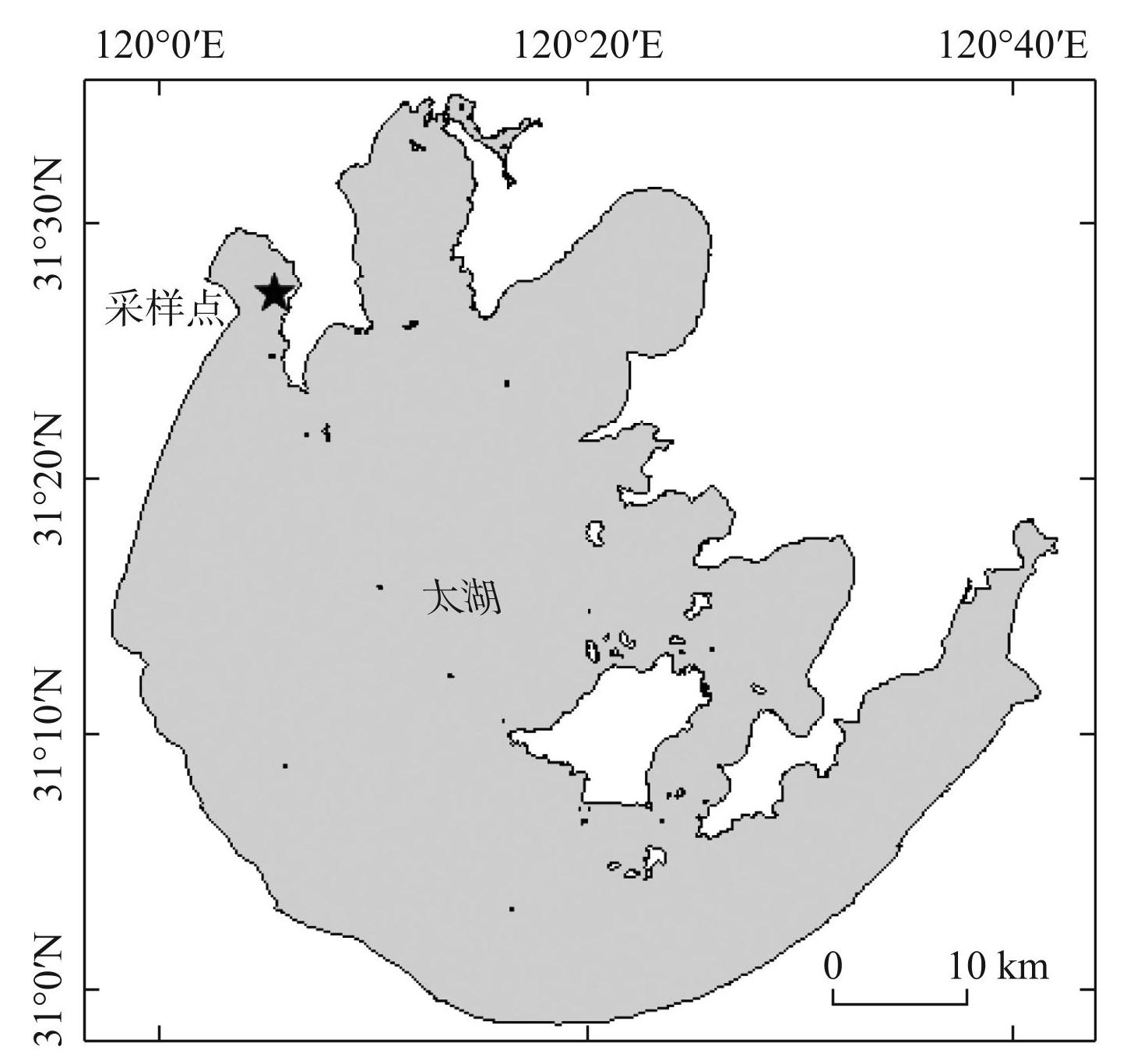
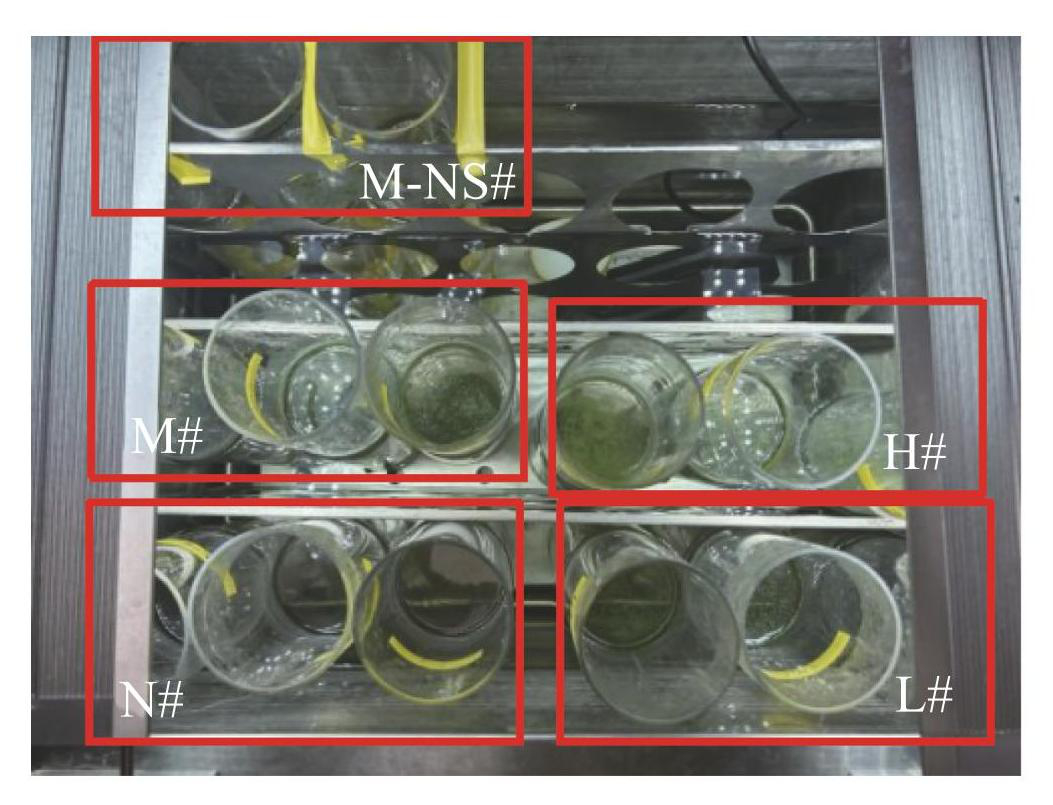
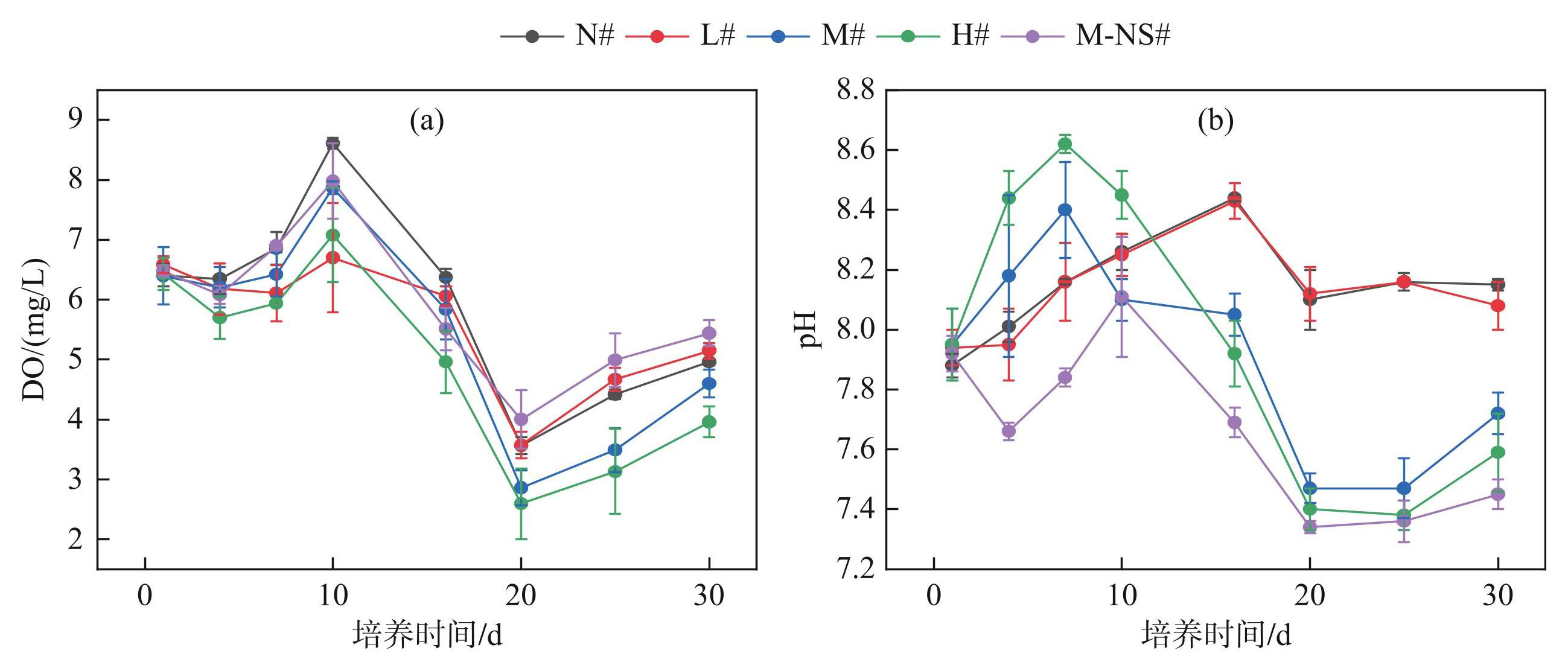
图3不同蓝藻添加量对上覆水DO(a)和pH(b)的影响
Fig.3Effects of different cyanobacteria additions on DO (a) and pH (b) in overlying water
下载:
全尺寸图片
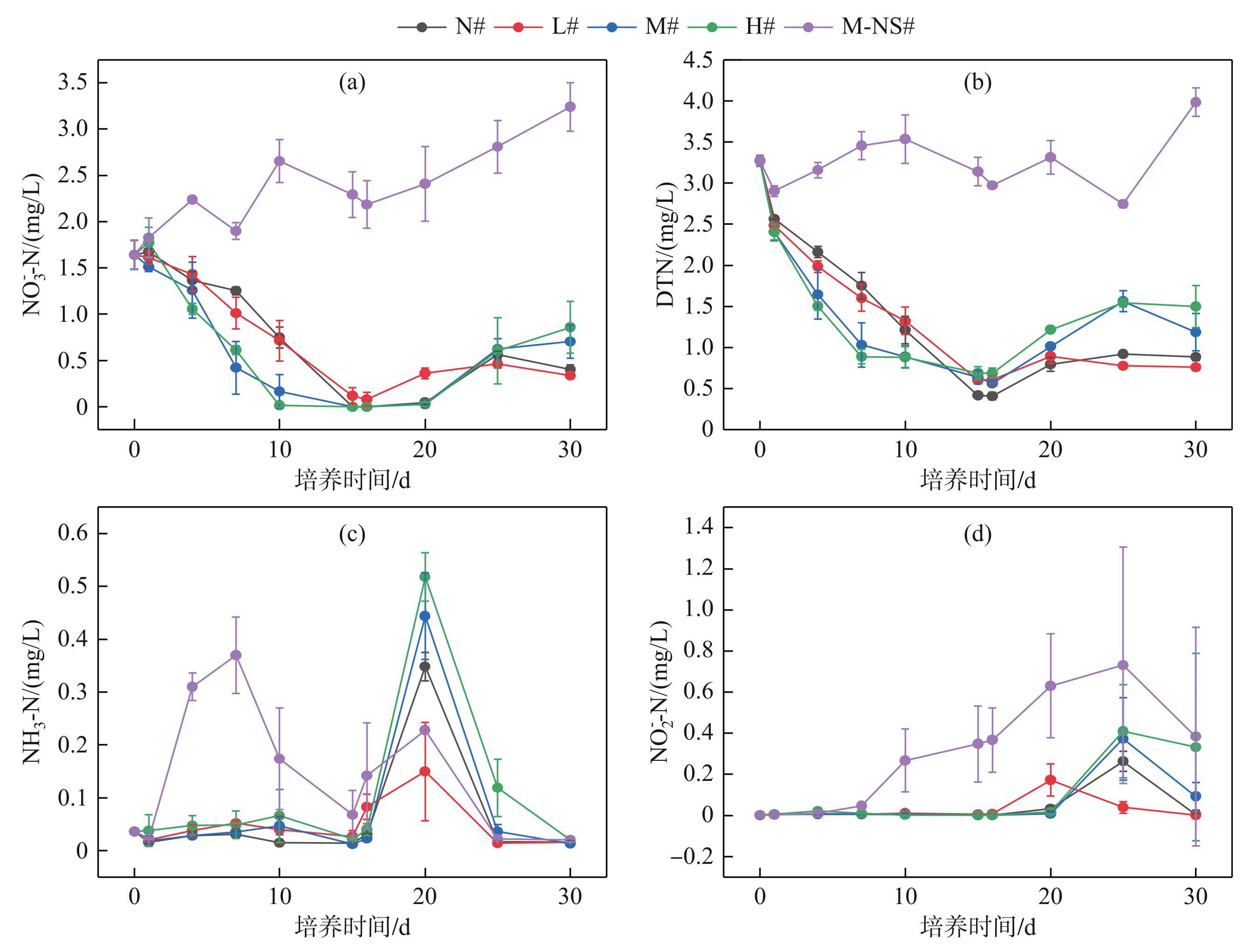
图4不同蓝藻添加量对上覆水氮浓度的影响
Fig.4Effects of different cyanobacteria additions on nitrogen concentration in overlying water
下载:
全尺寸图片
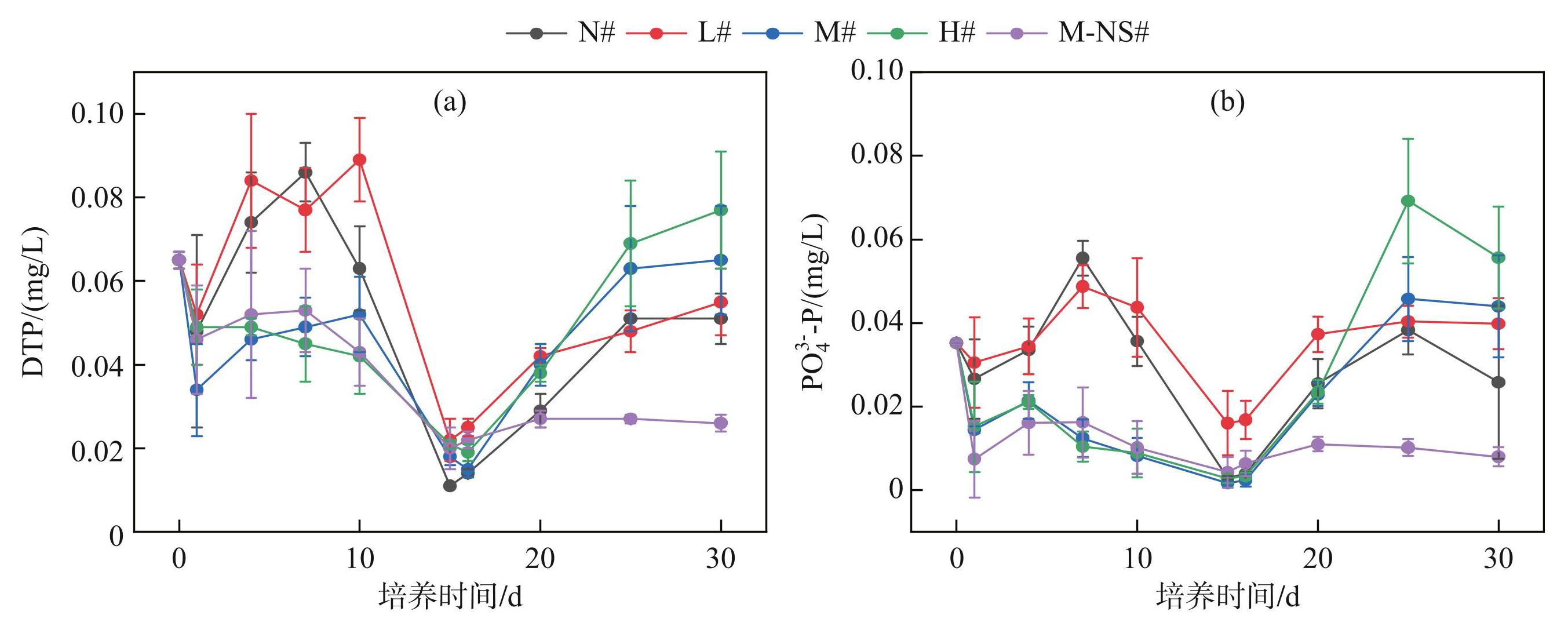
图5不同蓝藻添加量对上覆水磷浓度的影响
Fig.5Effects of different cyanobacteria additions on phosphorus concentration in overlying water
下载:
全尺寸图片
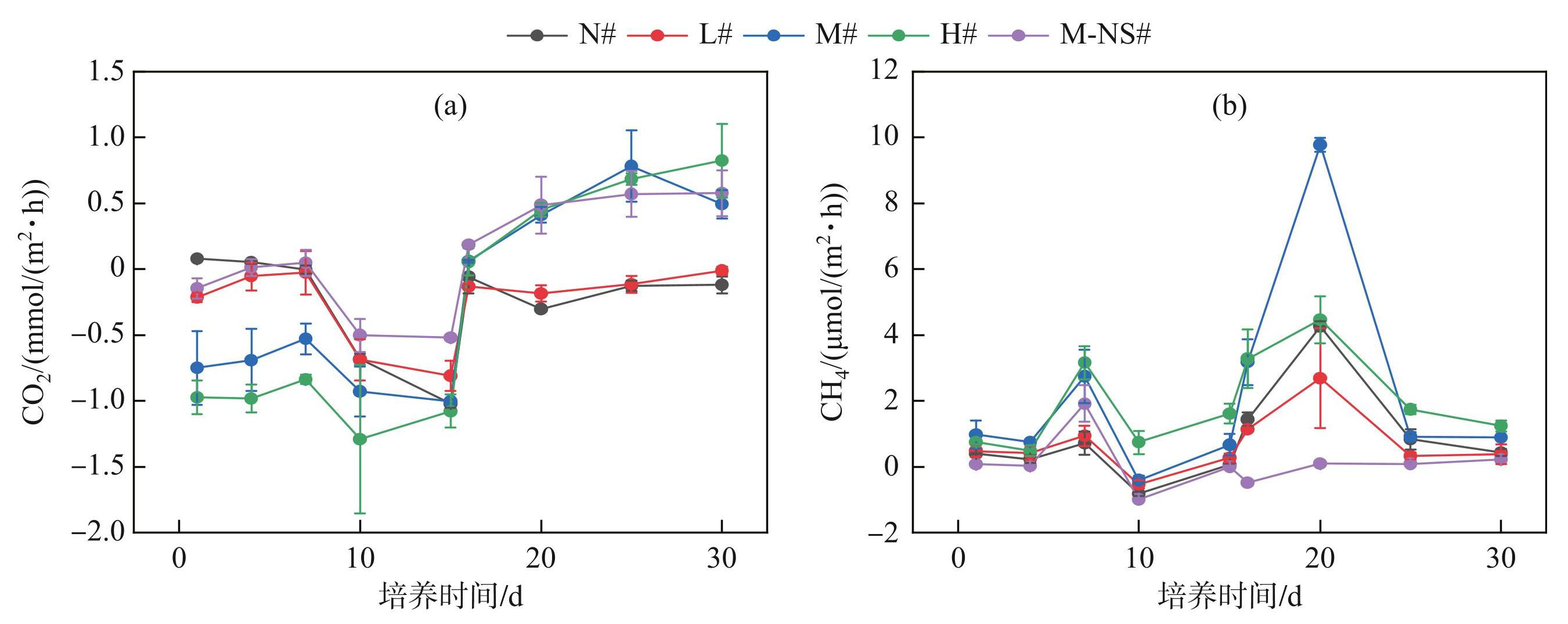
图6不同蓝藻添加量对沉积物CO2(a)和CH4(a)排放通量的影响
Fig.6Effects of different cyanobacteria additions on CO2(a) and CH4(b) emission fluxes in sediment
下载:
全尺寸图片
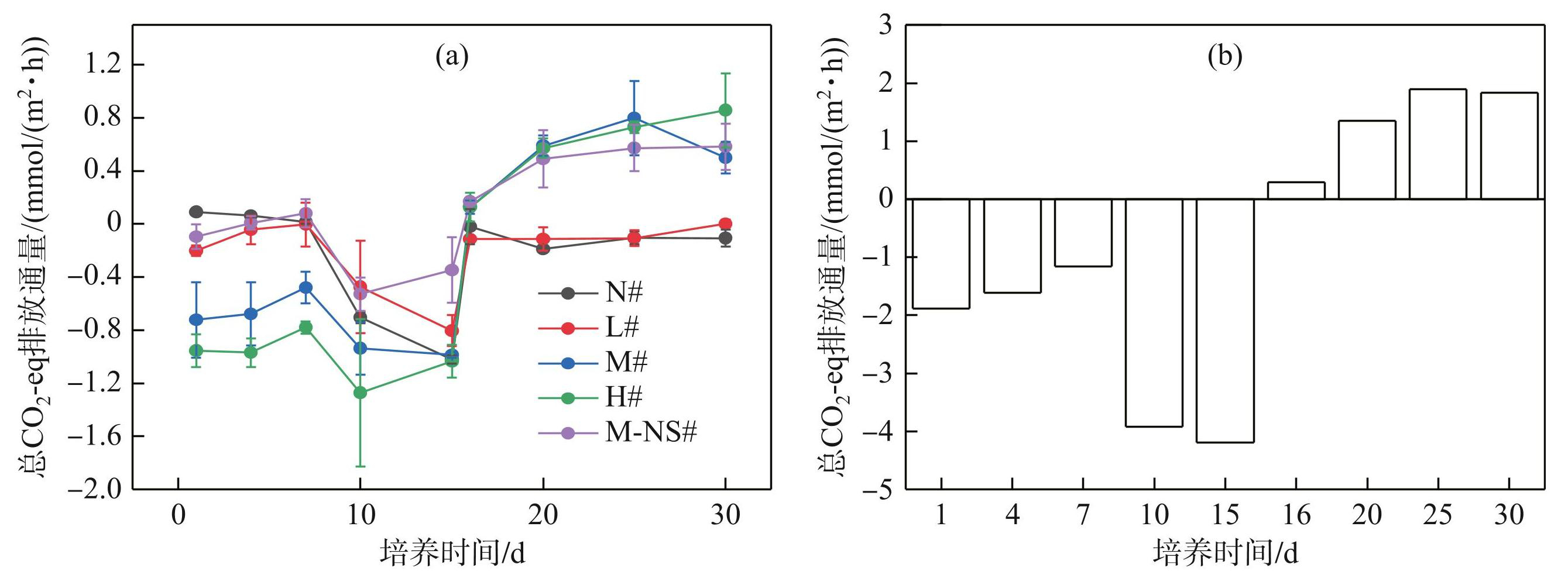
图7不同蓝藻添加量对沉积物总增温潜势的影响
Fig.7Effects of different cyanobacteria additions on the total warming potential in sediment
下载:
全尺寸图片
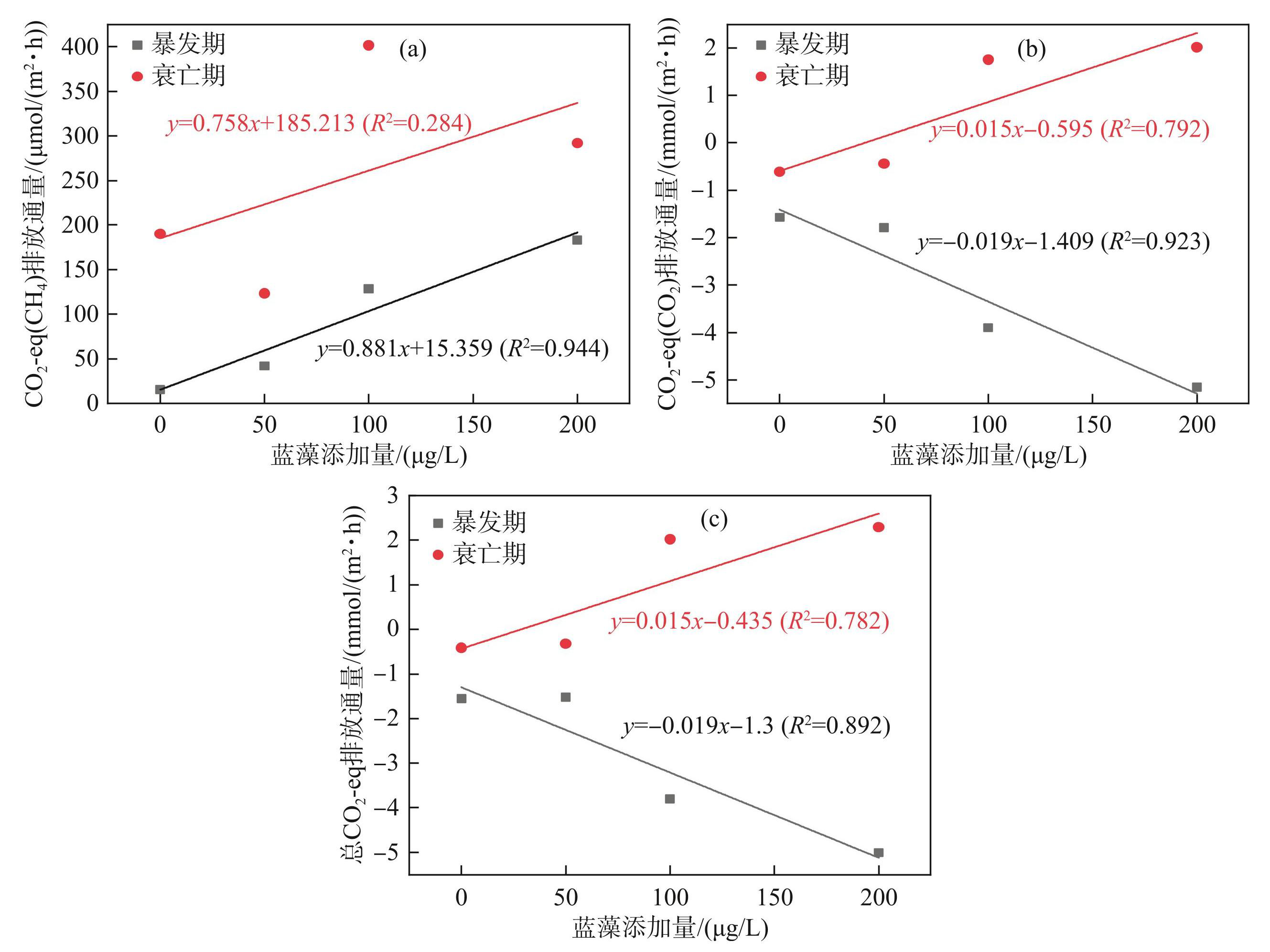
图8不同蓝藻添加量对沉积物CH4、CO2及总增温潜势的影响
Fig.8Effects of different cyanobacteria additions on CH4, CO2 and total warming potential in sediment
下载:
全尺寸图片
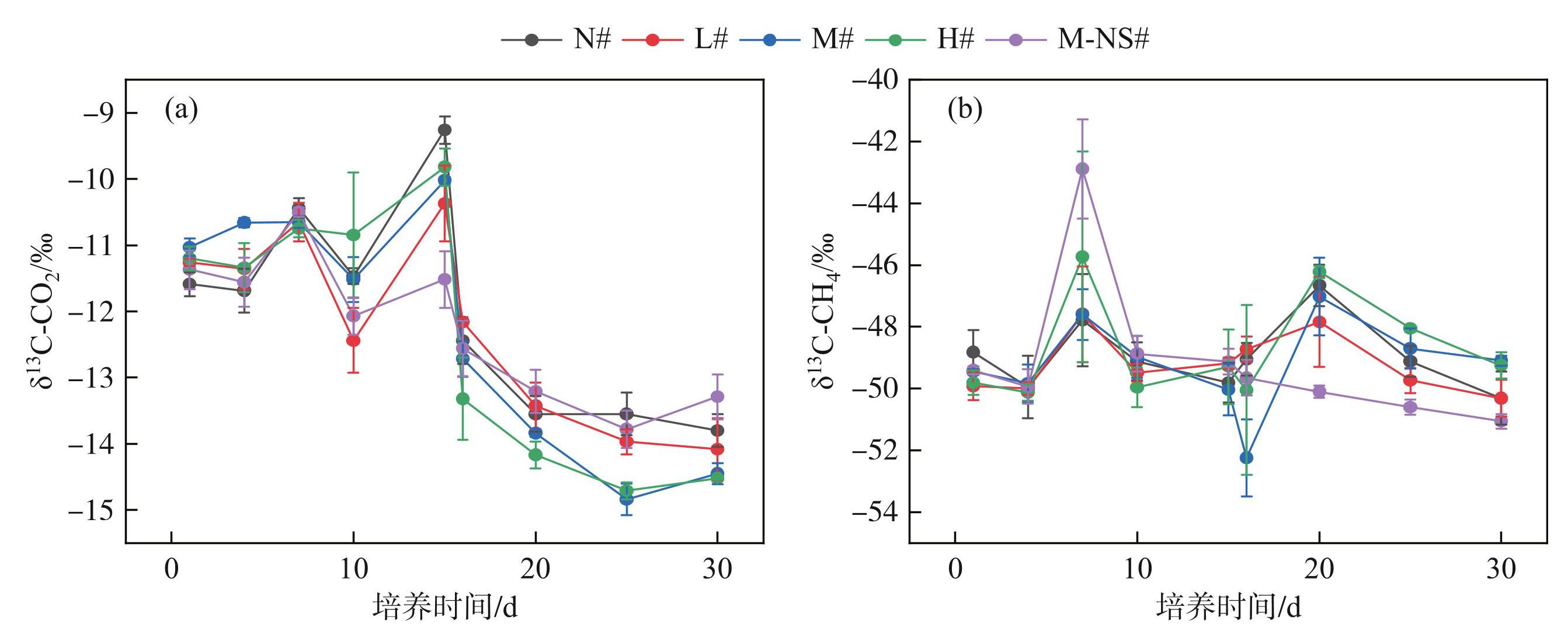
图9不同蓝藻添加量对沉积物δ13C-CO2和δ13C-CH4的影响
Fig.9Effects of different cyanobacteria additions on δ13C-CO2 and δ13C-CH4 in sediment
下载:
全尺寸图片
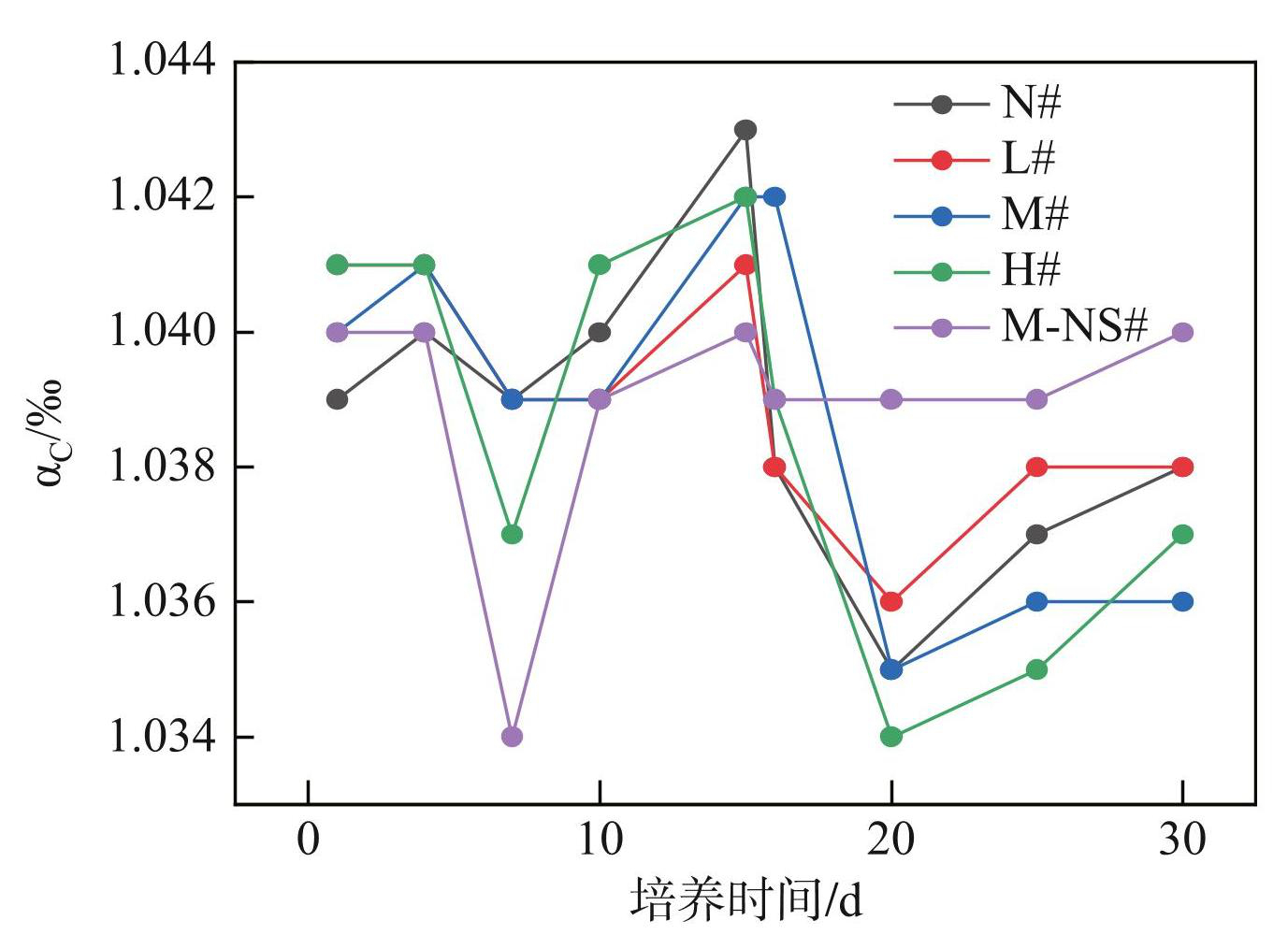
图10不同蓝藻添加量对沉积物CO2和 CH4表观分馏因子的影响
Fig.10Effects of different cyanobacteria additions on apparent fractionation factor of CO2 and CH4 in sediment
下载:
全尺寸图片
[1] |
Sobek S, Tranvik LJ, Prairie YT et al. Patterns and regulation of dissolved organic carbon: An analysis of 7,500 widely distributed lakes. Limnology and Oceanography,2007,52(3):1208-1219. DOI:10.4319/lo.2007.52.3.1208.
|
[2] |
Raymond PA, Hartmann J, Lauerwald R et al. Global carbon dioxide emissions from inland waters. Nature,2013,503(7476):355-359. DOI:10.1038/nature12760.
|
[3] |
Drake TW, Raymond PA, Spencer RGM. Terrestrial carbon inputs to inland waters: A current synthesis of estimates and uncertainty. Limnology and Oceanography Letters,2018,3(3):132-142. DOI:10.1002/lol2.10055.
|
[4] |
Xiao QT, Xu XF, Duan HT et al. Eutrophic Lake Taihu as a significant CO2 source during 2000-2015. Water Research,2020,170:115331. DOI:10.1016/j.watres.2019.115331.
|
[5] |
Hu WP, Jørgensen SE, Zhang FB et al. A model on the carbon cycling in Lake Taihu, China. Ecological Modelling,2011,222(16):2973-2991. DOI:10.1016/j.ecolmodel.2011.04.018.
|
[6] |
Meybeck M. Riverine transport of atmospheric carbon: Sources,global typology and budget. Water Air and Soil Pollution,1993,70(1):443-463. DOI:10.1007/BF01105015.
|
[7] |
Qi TC, Xiao QT, Miao YQ et al. Temporal and spatial variation of carbon dioxide concentration and its exchange fluxes in Lake Chaohu. J Lake Sci,2019,31(3):766-778. DOI:10.18307/2019.0315.[齐天赐, 肖启涛, 苗雨青等. 巢湖水体二氧化碳浓度时空分布特征及其水-气交换通量. 湖泊科学,2019,31(3):766-778.]
|
[8] |
Davidson TA, Audet J, Svenning JC et al. Eutrophication effects on greenhouse gas fluxes from shallow-lake mesocosms override those of climate warming. Global Change Biology,2015,21(12):4449-4463. DOI:10.1111/gcb.13062.
|
[9] |
McTigue ND, Walker QA, Currin CA. Refining estimates of greenhouse gas emissions from salt marsh“blue carbon” erosion and decomposition. Frontiers in Marine Science,2021,8:661442. DOI:10.3389/fmars.2021.661442.
|
[10] |
Li XJ, Xu XG, Zhao YP et al. Effects of algae and hydrophytes on the inorganic phosphorus pool of shallow hypereutrophic Lake Taihu. Water Supply,2022,22(1):181-194. DOI:10.2166/ws.2021.276.
|
[11] |
先宇. 水相CO2浓度对水华优势藻生长及固碳能力的影响[学位论文]. 重庆: 重庆大学,2021.
|
[12] |
Xing YP, Xie P, Yang H et al. Methane and carbon dioxide fluxes from a shallow hypereutrophic subtropical Lake in China. Atmospheric Environment,2005,39(30):5532-5540. DOI:10.1016/j.atmosenv.2005.06.010.
|
[13] |
Rudorff CM, Melack JM, MacIntyre S et al. Seasonal and spatial variability of CO2 emission from a large floodplain lake in the lower Amazon. Journal of Geophysical Research: Biogeosciences,2011,116(G4): G04007. DOI:10.1029/2011JG001699.
|
[14] |
Shao CL, Chen JQ, Stepien CA et al. Diurnal to annual changes in latent,sensible heat,and CO2 fluxes over a Laurentian Great Lake: A case study in western Lake Erie. Journal of Geophysical Research: Biogeosciences,2015,120(8):1587-1604. DOI:10.1002/2015JG003025.
|
[15] |
Boucher O, Randall D, Artaxo P et al. Contribution of working group I to the fifth assessment report of the intergovernmental panel on climate change. Climate Change,2013,5.
|
[16] |
Verpoorter C, Kutser T, Seekell DA et al. A global inventory of lakes based on high-resolution satellite imagery. Geophysical Research Letters,2014,41(18):6396-6402. DOI:10.1002/2014GL060641.
|
[17] |
Townsend-Small A, Disbennett D, Fernandez JM et al. Quantifying emissions of methane derived from anaerobic organic matter respiration and natural gas extraction in Lake Erie. Limnology and Oceanography,2016,61(S1): S356-S366. DOI:10.1002/lno.10273.
|
[18] |
Wang ZH, Cui FY, An Q et al. Study on influence of pH on the advance of eutrophication in reservoir. Water & Wastewater Engineering,2004,40(5):37-41.[王志红, 崔福义, 安全等.pH与水库水富营养化进程的相关性研究. 给水排水,2004,40(5):37-41.]
|
[19] |
Schulz S, Conrad R. Effect of algal deposition on acetate and methane concentrations in the profundal sediment of a deep lake(Lake Constance). FEMS Microbiology Ecology,1995,16(4):251-260. DOI:10.1111/j.1574-6941.1995.tb00289.x.
|
[20] |
Zhou CQ, Peng Y, Yu MT et al. Severe cyanobacteria accumulation potentially induces methylotrophic methane producing pathway in eutrophic lakes. Environmental Pollution,2022,292:118443. DOI:10.1016/j.envpol.2021.118443.
|
[21] |
Guillemette F, Leigh McCallister S, Del Giorgio PA. Selective consumption and metabolic allocation of terrestrial and algal carbon determine allochthony in lake bacteria. The ISME Journal,2016,10(6):1373-1382. DOI:10.1038/ismej.2015.215.
|
[22] |
Bartosiewicz M, Maranger R, Przytulska A et al. Effects of phytoplankton blooms on fluxes and emissions of greenhouse gases in a eutrophic lake. Water Research,2021,196:116985. DOI:10.1016/j.watres.2021.116985.
|
[23] |
Zhang L, Liu C, He K et al. Dramatic temporal variations in methane levels in black bloom prone areas of a shallow eutrophic lake. Science of the Total Environment,2021,767:144868. DOI:10.1016/j.scitotenv.2020.144868.
|
[24] |
Preheim SP, Olesen SW, Spencer SJ et al. Surveys,simulation and single-cell assays relate function and phylogeny in a lake ecosystem. Nature Microbiology,2016,1(9):16130. DOI:10.1038/nmicrobiol.2016.130.
|
[25] |
Qin BQ, Wang XD, Tang XM et al. Drinking water crisis caused by eutrophication and cyanobacterial bloom in Lake Taihu: Cause and measurement. Advances in Earth Science,2007,22(9):896-906. DOI:10.3321/j.issn:1001-8166.2007.09.003.[秦伯强, 王小冬, 汤祥明等. 太湖富营养化与蓝藻水华引起的饮用水危机——原因与对策. 地球科学进展,2007,22(9):896-906.]
|
[26] |
Wang SC, Sun PF, Zhang GB et al. Contribution of periphytic biofilm of paddy soils to carbon dioxide fixation and methane emissions. The Innovation,2022,3(1):100192. DOI:10.1016/j.xinn.2021.100192.
|
[27] |
Whiticar MJ, Faber E, Schoell M. Biogenic methane formation in marine and freshwater environments: CO2 reduction vs.acetate fermentation—Isotope evidence. Geochimica et Cosmochimica Acta,1986,50(5):693-709. DOI:10.1016/0016-7037(86)90346-7.
|
[28] |
Zhang L, He K, Wang T et al. Frequent algal blooms dramatically increase methane while decrease carbon dioxide in a shallow lake bay. Environmental Pollution,2022,312:120061. DOI:10.1016/j.envpol.2022.120061.
|
[29] |
Tang Q, Xue XF, Wang H et al. New knowledge of methanogens and methanotrophs in lake ecosystems. J Lake Sci,2018,30(3):597-610. DOI:10.18307/2018.0302.[唐千, 薛校风, 王惠等. 湖泊生态系统产甲烷与甲烷氧化微生物研究进展. 湖泊科学,2018,30(3):597-610.]
|
[30] |
Yan XC, Xu XG, Wang MY et al. Climate warming and cyanobacteria blooms: Looks at their relationships from a new perspective. Water Research,2017,125:449-457. DOI:10.1016/j.watres.2017.09.008.
|
[31] |
Gelesh L, Marshall K, Boicourt W et al. Methane concentrations increase in bottom waters during summertime Anoxia in the highly eutrophic estuary, Chesapeake Bay, U. S. A. Limnology and Oceanography,2016,61(S1): S253-S266. DOI:10.1002/lno.10272.
|
[32] |
Shi WQ, Pan G, Chen QW et al. Hypoxia remediation and methane emission manipulation using surface oxygen nanobubbles. Environmental Science & Technology,2018,52(15):8712-8717. DOI:10.1021/acs.est.8b02320.
|
[33] |
Encinas Fernández J, Peeters F, Hofmann H. Importance of the autumn overturn and anoxic conditions in the hypolimnion for the annual methane emissions from a temperate lake. Environmental Science & Technology,2014,48(13):7297-7304. DOI:10.1021/es4056164.
|
[34] |
Prasanna R, Kumar V, Kumar S et al. Methane production in rice soil is inhibited by cyanobacteria. Microbiological Research,2002,157(1):1-6. DOI:10.1078/0944-5013-00124.
|
[35] |
Liu XH, Gao Y, Zhang ZH et al. Sediment-water methane flux in a eutrophic pond and primary influential factors at different time scales. Water,2017,9(8):601. DOI:10.3390/w9080601.
|
[36] |
Cao YY. Study on the relationship between chlorophyll a content and pH, DO,nitrogen and phosphorus concentrations—Taking Yangxi Reservoir as an example. Modern Business Trade Industry,2015,36(17):208-209.[曹樱樱. 叶绿素a含量与pH、DO、氮磷浓度关系研究——以杨溪水库为例. 现代商贸工业,2015,36(17):208-209.]
|
[37] |
Zhang JY, Huang J, Yan F et al. Preliminary study on characters of dissolved oxygen and the relationship with pH in Meiliang Lake. Journal of Fudan University: Natural Science,2009,48(5):623-627.[张军毅, 黄君, 严飞等. 梅梁湖水体溶解氧特征及其与pH的关系分析. 复旦学报: 自然科学版,2009,48(5):623-627.]
|
[38] |
You L, Cui LF, Liu ZW et al. Correlation analysis of parameters in algal growth. Environmental Science & Technology,2007,30(9):42-44,117.[游亮, 崔莉凤, 刘载文等. 藻类生长过程中DO、pH与叶绿素相关性分析. 环境科学与技术,2007,30(9):42-44,117.]
|
[39] |
Borrel G, Jézéquel D, Biderre-Petit C et al. Production and consumption of methane in freshwater lake ecosystems. Research in Microbiology,2011,162(9):832-847. DOI:10.1016/j.resmic.2011.06.004.
|
[40] |
Maruya Y, Nakayama K, Sasaki M et al. Effect of dissolved oxygen on methane production from bottom sediment in a eutrophic stratified lake. Journal of Environmental Sciences,2023,125:61-72. DOI:10.1016/j.jes.2022.01.025.
|
[41] |
Chan CN, Shi HY, Liu BY et al. CO2 and CH4 emissions from an arid fluvial network on the Chinese Loess Plateau. Water,2021,13(12):1614. DOI:10.3390/w13121614.
|
[42] |
Wang X, Li ZC, Li X et al. Effect of the decline of cyanobacterial bloom on the organic carbon composition of lake sediment carbon pool. J Lake Sci,2020,32(2):346-356. DOI:10.18307/2020.0212.[汪旋, 李致春, 李翔等. 蓝藻水华衰亡对沉积物碳库有机碳组分的影响. 湖泊科学,2020,32(2):346-356.]
|
[43] |
Wang HJ, Lu JW, Wang WD et al. Methane fluxes from the littoral zone of hypereutrophic Taihu Lake, China. Journal of Geophysical Research: Atmospheres,2006,111(D17): D17109. DOI:10.1029/2005JD006864.
|
[44] |
Zhang N, He K, Zhong JC et al. Enhancement and mechanism of algal-derived organic matter deposition on lake sediment methane release. China Environmental Science,2023,43(12):6641-6650.[张楠, 何凯, 钟继承等. 藻源性有机质沉降对沉积物甲烷释放促进作用. 中国环境科学,2023,43(12):6641-6650.]
|
[45] |
Dong XH, Anderson NJ, Yang XD et al. Carbon burial by shallow lakes on the Yangtze floodplain and its relevance to regional carbon sequestration. Global Change Biology,2012,18(7):2205-2217. DOI:10.1111/j.1365-2486.2012.02697.x.
|
[46] |
Lamarche-Gagnon G, Wadham JL, Sherwood Lollar B et al. Greenland melt drives continuous export of methane from the ice-sheet bed. Nature,2019,565(7737):73-77. DOI:10.1038/s41586-018-0800-0.
|
[47] |
Michaud AB, Dore JE, Achberger AM et al. Microbial oxidation as a methane sink beneath the west Antarctic ice sheet. Nature Geoscience,2017,10:582-586. DOI:10.1038/ngeo2992.
|
[48] |
Pain AJ, Martin JB, Martin EE et al. Heterogeneous CO2 and CH4 content of glacial meltwater from the Greenland Ice Sheet and implications for subglacial carbon processes. The Cryosphere,2021,15(3):1627-1644. DOI:10.5194/tc-15-1627-2021.
|
[49] |
Bucha M, Jędrysek MO, Kufka D et al. Methanogenic fermentation of lignite with carbon-bearing additives,inferred from stable carbon and hydrogen isotopes. International Journal of Coal Geology,2018,186:65-79. DOI:10.1016/j.coal.2017.11.020.
|
[50] |
Hornibrook ERC, Longstaffe FJ, Fyfe WS. Evolution of stable carbon isotope compositions for methane and carbon dioxide in freshwater wetlands and other anaerobic environments. Geochimica et Cosmochimica Acta,2000,64(6):1013-1027. DOI:10.1016/S0016-7037(99)00321-X.
|
[51] |
刘喜乐. 褐煤与秸秆协同产甲烷过程中生化代谢及同位素特征研究[学位论文]. 北京: 中国矿业大学,2022.
|
[52] |
Frumin GT, Gildeeva IM. Eutrophication of water bodies—A global environmental problem. Russian Journal of General Chemistry,2014,84(13):2483-2488. DOI:10.1134/S1070363214130015.
|
[53] |
Wik M, Varner RK, Anthony KW et al. Climate-sensitive northern lakes and ponds are critical components of methane release. Nature Geoscience,2016,9:99-105. DOI:10.1038/ngeo2578.
|
[54] |
Yin HB, Yun Y, Zhang YL et al. Phosphate removal from wastewaters by a naturally occurring,calcium-rich sepiolite. Journal of Hazardous Materials,2011,198:362-369. DOI:10.1016/j.jhazmat.2011.10.072.
|
[55] |
Wu F, Zhan JY, Deng XZ et al. Influencing factors of lake eutrophication in China—A case study in 22 lakes in China. Ecology and Environmental Sciences,2012,21(1):94-100.[吴锋, 战金艳, 邓祥征等. 中国湖泊富营养化影响因素研究——基于中国22个湖泊实证分析. 生态环境学报,2012,21(1):94-100.]
|
[56] |
Chen C, Zhong JC, Fan CX et al. Simulation research on the release of internal nutrients affected by different dredging methods in lake. Environmental Science,2013,34(10):3872-3878.[陈超, 钟继承, 范成新等. 湖泊疏浚方式对内源释放影响的模拟研究. 环境科学,2013,34(10):3872-3878.]
|
[57] |
Zhang YY, Yan SH, Liu HQ et al. Mechanism of interaction between Eichhornia crassipes and phosphorus in ecological-remediation technology of eutrophic water. Ecology and Environmental Sciences,2017,26(4):721-728.[张迎颖, 严少华, 刘海琴等. 富营养化水体生态修复技术中凤眼莲与磷素的互作机制. 生态环境学报,2017,26(4):721-728.]
|