摘要
淡水养殖池塘溶解性有机质(DOM)的光化学过程具有重要的环境效应,其具体过程尚不清楚。本文利用光谱和分子探针技术探究太湖流域2个典型淡水养殖池塘(中华绒螯蟹塘和克氏原鳌虾塘)DOM在不同光辐照强度下的光化学行为。通过紫外吸收光谱和三维荧光光谱技术揭示了养殖池塘DOM组分性质随光辐照时间的变化过程,分子探针技术定量DOM光化学过程产生的光敏活性中间体(PPRIs)——3DOM*、1O2和·OH的生成速率、稳态浓度和量子产率。结果表明,淡水养殖池塘DOM可分离出2个类腐殖质组分(C1和C2)和1个类蛋白组分(C3),淡水养殖池塘DOM腐殖化程度低,强自生源特征明显,兼具陆源与内源属性。中华绒螯蟹塘溶解性有机碳浓度和富营养化程度以及DOM各荧光组分强度均高于克氏原鳌虾塘。淡水养殖池塘DOM的组分性质在不同光辐照强度下的变化存在差异。低光辐照强度下,DOM各组分性质均无明显变化,但高光辐照强度下,淡水养殖池塘DOM的荧光强度、分子量、芳香性和疏水性随辐照时间的增加而下降。中华绒螯蟹塘DOM中C1、C2和C3荧光组分的光降解速率分别为0.014、0.007和0.021 h-1,均高于克氏原鳌虾塘DOM对应组分光降解速率(0.012、0.004和0.02 h-1),C3组分占比小但表现出更强的光化学活性。PPRIs的生成潜能随着光辐照强度的增加而增加,中华绒螯蟹塘DOM在高光辐照强度下3DOM*、1O2和·OH生成速率分别为1.41×10-8、1.32×10-8和5.52×10-12 mol/(L·s),高于克氏原鳌虾塘DOM对应PPRIs的生成速率(9.77×10-9、6.34×10-9和2.50×10-12 mol/(L·s))。本研究为丰富淡水养殖池塘DOM环境行为机制和防控养殖池塘有机污染物提供理论支撑。
Abstract
The photochemical processes of dissolved organic matter (DOM) in freshwater aquaculture ponds have significant environmental effects, yet the specific mechanisms remain unknown. This study investigated the photochemical behaviors of DOM in two typical freshwater aquaculture ponds (Eriocheir sinensis pond and Procambarus clarkii pond) in the Taihu Basin under varying light irradiation intensities using spectroscopic and molecular probe techniques. UV absorption and three-dimensional fluorescence spectroscopy were employed to elucidate changes in the properties of DOM components derived from freshwater aquaculture ponds during light exposure, while molecular probe techniques quantified production rates, steady-state concentrations, and quantum yields of photochemically produced reactive intermediates (PPRIs) (3DOM*, 1O2 and ·OH) generated by the photochemical processes of DOM. Results revealed that two humus-like fractions (C1 and C2) as well as one protein-like fraction (C3) could be modeled from DOM in freshwater aquaculture ponds, which exhibited low degrees of humification but distinct characteristics associated with strong autochthonous sources along with terrestrial and endogenous origins. Dissolved organic carbon concentration, eutrophication degree, and intensity of each fluorescent component were higher in Eriocheir sinensis pond compared to Procambarus clarkii pond. Furthermore, properties of DOM components varied under different light irradiance intensities; no significant changes occurred at low intensities, whereas fluorescence intensity, molecular weight, aromaticity, hydrophobicity declined with increasing irradiation time at high intensities. The photodegradation rates of C1, C2, and C3 of DOM from the Eriocheir sinensis pond were 0.014, 0.007 and 0.021 h-1, respectively, higher than the corresponding components in DOM from Procambarus clarkii pond (0.012, 0.004 and 0.02 h-1). Notably, the C3 component exhibited a relatively small proportion but displayed stronger photochemical activity. The production potential of PPRIs increased with the increment of light irradiation intensity. Moreover, the production rates of 3DOM*, 1O2, and ·OH were measured as 1.41×10-8, 1.32×10-8, 5.52×10-12 mol/(L·s), respectively, at high light irradiation intensities in the Eriocheir sinensis pond, higher than the corresponding PPRIs production rates for DOM in Procambarus clarkii pond (9.77×10-9, 6.34×10-9, 2.50×10-12 mol/(L·s)). This study provided theoretical support for enriching the mechanisms of DOM environmental behavior in freshwater aquaculture ponds and preventing and controlling organic pollutants in aquaculture ponds.
溶解性有机质(DOM)是指能够通过0.45 μm滤膜,包含多种天然生物分子,如脂类、肽/蛋白质、氨基酸、糖类、木质素、单宁酸等十分复杂的有机质混合物[1]。DOM可影响重金属、有机污染物的迁移转化、生物利用性及毒性[2-3],同时也是全球重要的有机碳库[4]。我国淡水养殖池塘总面积达257万hm2[5],水产养殖产量居世界首位。由于高密度、集约化养殖,剩余饵料、水产品排泄物导致淡水养殖池塘DOM普遍偏高[6]。DOM在水环境中会发生一系列环境行为(吸附-解吸、络合、光转化和生物降解等)[7-8],特别在水体表层,太阳光作用下DOM会发生光化学过程,此类过程可降低溶解性有机碳(DOC)含量、分子量、芳香性和腐殖化程度,从而改变DOM的生物可利用性,影响水环境中碳和营养物质循环[9-11]。光降解产物中的小分子量DOM可以作为藻类的营养来源,刺激藻的生长,进而影响养殖水体中水生生物繁殖[12]。此外,DOM光化学过程中会产生光敏活性中间体(PPRIs),如三重激发态DOM(3DOM*)、单线态氧(1O2)和羟基自由基(·OH)等[13]。这些活性物质虽瞬时产生且微量,但是氧化性很强[14-15],可显著地影响养殖池塘中有机污染物迁移转化[16-18],如养殖池塘抗生素在PPRIs的作用下会发生间接光解[19]。PPRIs还会对水生生物产生氧化刺激,如PPRIs会引起蓝藻中脂质氧化和DNA链断裂,甚至抑制光合作用蛋白质的重新合成,进而抑制蓝藻的生长[20]。目前关于淡水养殖池塘DOM的研究不多,主要涉及其组分性质,如Wang等[21]发现中国东部淡水养殖池塘DOM主要是由陆地输入的腐殖酸/黄腐酸以及来自饲料颗粒和鱼类活动的色氨酸/酪氨酸类蛋白组成;Ryan等[22]发现养殖尾水DOM富含色氨酸类蛋白荧光成分,其生物降解性增加了受纳水体异养性,但淡水养殖池塘DOM的光化学过程鲜有报道。
光谱技术是研究DOM光化学过程的重要手段,紫外可见光谱(UV-Vis)、三维荧光光谱结合平行因子分析法(EEMs-PARAFAC)因具备需要样品体积少、效率高、检出限低、不破坏样品结构、获取信息量大等优点,被广泛应用于DOM光化学过程研究[23-24]。有研究者利用UV-Vis和EEMs-PARAFAC技术发现陆源类腐殖质和色氨酸类蛋白组分强烈促进PPRIs的光化学形成[25]。PPRIs检测方法主要包括顺磁共振法[26]、荧光法[27]、分子探针等,但顺磁共振法、荧光法耗时长、灵敏度较低,无法反映PPRIs的实时生成,而分子探针具有快速、灵敏和低毒性的优点已广泛用于PPRIs的定量[28-29],如2,4,6-三甲基苯酚(TMP)和糠醇(FFA)可作检测3DOM*和1O2的分子探针,苯和对苯二甲酸(TPA)可用作检测·OH的分子探针[15,30-31]。分子探针技术不仅可以对PPRIs定量,还可以利用所得结果与光谱指数的关系构建量子产率和稳态浓度预测模型[31-32]。
太阳光辐照强度是影响DOM光化学过程的关键因素之一,因此,本研究利用光谱(UV-Vis、EEMs-PARAFAC)和分子探针技术,探究不同光辐照强度下,典型淡水养殖池塘DOM的光化学过程中组分与性质的变化,同时利用分子探针技术量化3DOM*、1O2和·OH的生成速率( 、 和R·OH)、量子产率( 、 和Φ·OH)和稳态浓度([3DOM*]ss、[1O2]ss和[·OH]ss)。本研究可为深入理解淡水养殖池塘DOM光化学过程提供参考,并为淡水养殖池塘有机污染物防控提供理论支撑。
1 材料和方法
1.1 样品的采集与预处理
所用水样于2023年6月采自太湖流域常州市金坛区金城镇淡水水产养殖区,此区域水产养殖业发达,是江苏省重要的水产养殖区。分别采集3个位置相近的中华绒螯蟹塘(以下简称螃蟹塘;31°48′25.5″N,119°32′43.8″E)和克氏原螯虾塘(以下简称龙虾塘;31°48′26.17″N,119°33′4.79″E)表层水样后混合均匀,混合水样保存于酸洗的高密度聚乙烯瓶中,放入车载冰箱(4℃)4 h内运输到实验室,随后用0.45 μm的水系聚醚砜滤膜抽滤,过滤后的水样置于4℃冰箱中避光保存,待后续实验使用。螃蟹苗和龙虾苗放养时间为3月,螃蟹苗放养量为800~1200尾/666.67 m2,龙虾苗放养量为6000~7000尾/666.67 m2,采样时水产品活动频繁。
1.2 基本水化学指标测定
DOC采用总有机碳分析仪(TOC-L,Shimadzu)测定,总磷(TP)、总氮(TN)消解后以及硝态氮(NO-3-N)和亚硝态氮(NO-2-N)浓度都采用流动分析仪(Skalar,San++)测定,溶解氧(DO)、pH、电导率采用水质多参数仪(U-50,Horriba)测定,钙离子(Ca2+)、镁离子(Mg2+)和总铁(Fe)浓度采用电感耦合等离子体发射光谱仪(X-seriers,USA)检测,氟离子(F-)、氯离子(Cl-)、溴离子(Br-)浓度采用离子色谱仪(ICS1100,Thermo Fisher)测定。
1.3 DOM光化学实验
DOM光化学实验在光化学反应仪(HF-GHX-Ⅵ,贺帆)中进行,用290 nm滤光片截取290 nm以下的波段,模拟290~800 nm波段。为区分不同光强对DOM光化学过程的影响,用1000W氙灯调节不同光强,分别设置低光强(6.67 mW/cm2)和高光强(33.6 mW/cm2)模拟不同太阳光强度,290~800 nm波段低光强和高光强光谱图如附图Ⅰ所示,高光强全波段光辐照强度接近真实太阳光光辐照强度[33-34]。将50 mL的DOM溶液装入石英管中,使用循环冷却系统将温度控制在(25±0.5)℃,转速设为25 r/min,分别在0、2、4、6、8、12、16和24 h取样,分别进行DOC测定和UV-Vis、EEMs扫描。实验均设置3个平行。
采用TMP、FFA和TPA作为分子探针分别量化DOM光化学过程中生成3DOM*、1O2和·OH的潜能。通过测定TMP、FFA的减少量来量化3DOM*、1O2的生成速率、稳态浓度和量子产率,而·OH的生成速率、稳态浓度和量子产率则是通过2-羟基对苯二甲酸(hTPA)的生成来量化[31,35-36]。
1.4 光谱扫描及数据处理
UV-Vis由配备有1 cm石英比色皿的紫外可见分光光度计(UV-2700,Shimadzu)扫描获得,波长范围为200~800 nm,间隔为1 nm,以超纯水作为空白,采用700~800 nm的吸光度均值进行光谱校正。EEMs由激发光源为450 W氙灯,配备有CCD检测器的三维荧光光谱仪(Fluologic-3,HORIBA)扫描获得。以超纯水为空白,激发波长(Ex)范围为250~450 nm(5 nm间隔),发射波长(Em)范围为250~550 nm(1 nm间隔)。此外,由UV-Vis获得的光谱特征参数如相对分子质量比(E2∶E3)、芳香性指数(SUVA254)和由EEMs获得的光谱特征参数如腐殖化指数(HIX)、自生源指数(BIX)、荧光指数(FI)、惰性类腐殖质物质和不稳定类蛋白组分荧光强度比(Peak C/Peak T)可反映DOM光化学过程中性质的变化,对应的描述与计算方法如附表Ⅰ所示。
1.5 PPRIs的量化
1.5.1 探针化合物的检测
探针化合物TMP、FFA采用配备有紫外检测器的高效液相色谱仪(Agilent 1260)测定,hTPA采用配备有荧光检测器的高效液相色谱仪(Agilent LC-6AD)测定。具体检测参数如附表Ⅱ所示。
1.5.2 生成速率、量子产率和稳态浓度的计算
通过TMP的减少量对 、[3DOM*]ss和 量化,通过FFA的减少量对 、[1O2]ss和 量化,通过hTPA的生成量对R·OH、[·OH]ss和Φ·OH量化。对应的计算公式如附件Ⅰ所示。
1.6 数据处理
利用Matlab R2012a软件的drEEM工具箱消除瑞利散射峰和拉曼散射峰,通过drEEM和N-way工具箱对EEMs数据进行PARAFAC分析[37],本研究EEM数据矩阵数量为90个,满足PARAFAC数据处理的要求[38],用最大荧光强度Fmax(单位为R.U.)[39-40]代表DOM光化学过程中荧光组分丰度变化。使用 IBM SPSS Statistics 20 对数据进行独立样本t检验分析,P<0.05、P<0.01和P>0.05分别表示差异显著、极显著和不显著。
2 结果
2.1 基本水化学参数
DOC浓度可以评估水体中DOM浓度[41],由表1可知,螃蟹塘和龙虾塘的DOC浓度分别为11.35和4.73 mg/L,与龙虾塘相比,螃蟹塘具有更高的DOC浓度。螃蟹塘和龙虾塘pH值均大于9。与龙虾塘相比,螃蟹塘TN和TP浓度均远高于龙虾塘。养殖池塘DO浓度较高(约9 mg/L),满足虾蟹氧耗需求(4~5 mg/L)。螃蟹塘和龙虾塘水样主要阳离子均为Ca2+,浓度分别为27.26、27.38 mg/L,主要阴离子均为Cl-,浓度分别为65.11、56.54 mg/L(表1)。
表1淡水养殖池塘基本水质指标
Tab.1 Basic water quality indicators of freshwater aquaculture ponds

2.2 养殖池塘DOM光化学过程中光谱特征
2.2.1 EEMs-PARAFAC特征
利用PARAFAC解析养殖池塘DOM的EEMs数据,得到3个荧光组分,并与 Open Flour数据库(网址http://openfluor.lablicate.com)进行比对,结果如图1所示。其中C1组分(Ex/Em=250(305)/409 nm)与传统M峰相似,为微生物源类腐殖质荧光团,与微生物降解有关,广泛存在于淡水水体中[42-44]。C2组分(Ex/Em=255(360)/473 nm)与传统A峰相似,是具有较高芳香性和分子量大小的陆源类腐殖质物质[44-46]。C3组分(Ex/Em=280/330 nm)与传统T峰(色氨酸)相似,为类蛋白物质,并具有较高的生物利用度[47-49]。
淡水养殖池塘DOM光转化过程中,最大荧光强度变化如图2所示,螃蟹塘DOM三种荧光组分的初始Fmax值均大于龙虾塘。低光辐照强度下,除螃蟹塘C1组分Fmax值光辐照24 h后下降了0.34 R.U.外,螃蟹塘与龙虾塘DOM其余各荧光组分随辐照时间变化均不大,而高光辐照强度下,淡水养殖池塘DOM中C1、C2和C3组分的Fmax值均随时间增加呈显著下降趋势(P<0.01),其中螃蟹塘DOM中C1、C2和C3组分的Fmax值分别由5.42、2.81和1.86 R.U.降为3.84、2.36 和1.10 R.U.,龙虾塘DOM中C1、C2和C3组分的Fmax值分别由1.29、0.77和0.75 R.U.降为0.95、0.69和0.46 R.U.。
DOM荧光组分C1、C2、C3降解符合一级动力学,具体见附件Ⅱ,荧光强度光化学降解一级动力学表明,螃蟹塘DOM中C1、C2和C3组分光化学降解速率分别为0.014、0.007和0.021 h-1,龙虾塘DOM中C1、C2和C3组分光化学降解速率分别为0.012、0.004和0.020 h-1,尽管C3组分荧光强度最低,但光化学降解速率均最大(图3a,b)。DOM荧光组分光降解前后相对丰度变化如图3c所示,光降解前,螃蟹塘和龙虾塘DOM类腐殖质物质(C1+C2)占总荧光强度的比例分别为82%和73%,此外,两个养殖池塘DOM中C1组分相对丰度均最大,其占总荧光强度的比例分别为54%和46%。淡水养殖池塘DOM经过低光强光辐照24 h后,各荧光组分相对丰度无显著变化(P>0.05),而淡水养殖池塘DOM经过高光强光辐照24 h后,螃蟹塘和龙虾塘DOM的类腐殖质物质相对丰度增加,而类蛋白C3组分相对丰度则分别由18%、27%降为15%、22%。

图1由PARAFAC解析养殖池塘DOM EEMs所得荧光组分及对应荷载
Fig.1Analysis of fluorescence fractions and corresponding loads obtained from DOM EEMs in aquaculture ponds by PARAFAC
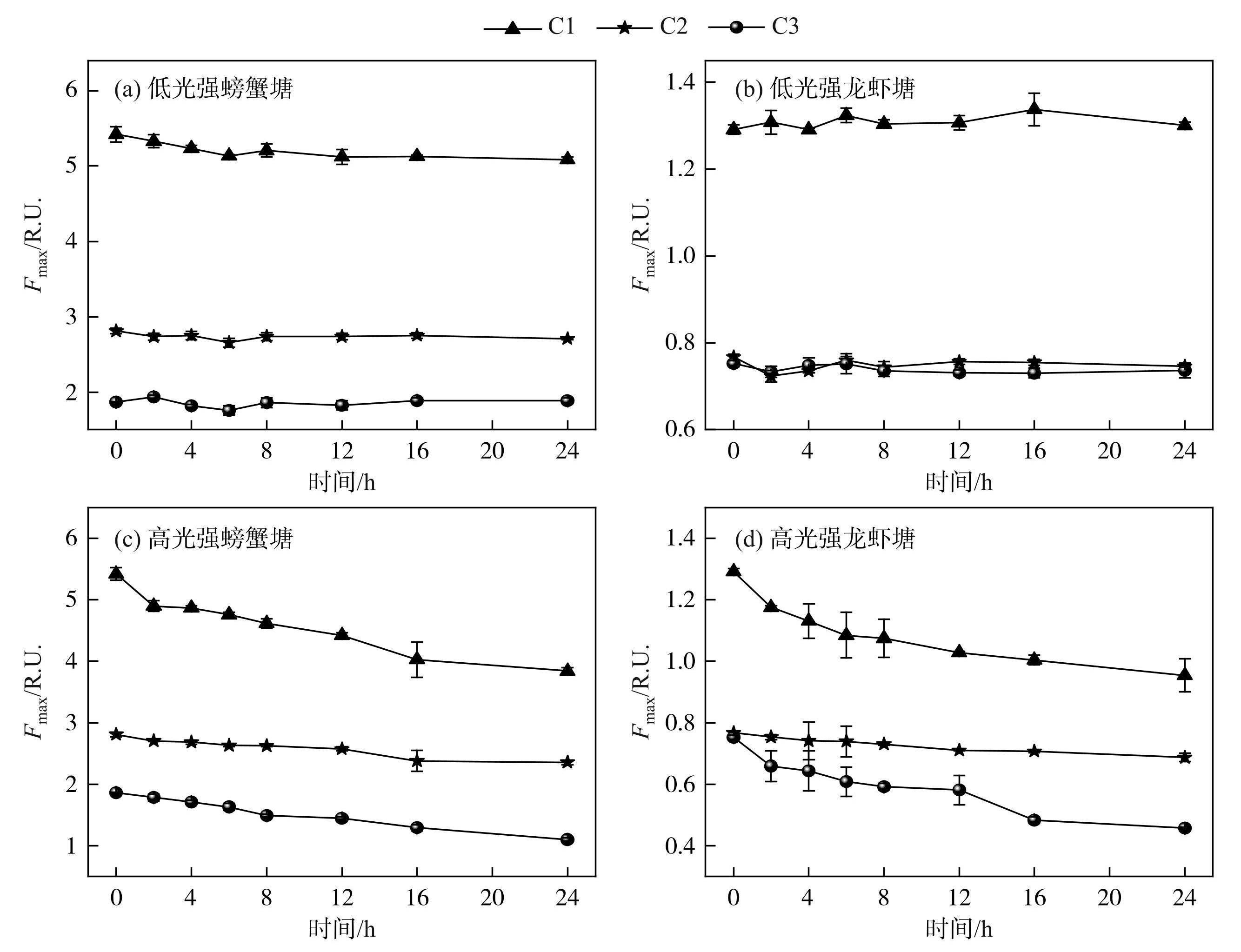
图2淡水养殖池塘DOM光转化过程中最大荧光强度变化
Fig.2Changes in maxmum fluorescence intensity during photoconversion of DOM in freshwater aquaculture ponds

图3淡水养殖池塘DOM光化学过程中荧光强度光化学降解一级动力学(a和b)和荧光强度相对丰度变化(c)
Fig.3First-order kinetics of photochemical degradation of fluorescence intensity (a and b) and changes in relative abundance of fluorescence intensity (c) during photoconversion of DOM in freshwater aquaculture ponds
2.2.2 淡水养殖池塘DOC浓度与DOM光谱参数特征变化
2个淡水养殖池塘光化学过程中DOC浓度与DOM光谱参数的变化如图4所示。光辐照过程中,淡水养殖池塘DOC浓度在一定范围内波动。螃蟹塘和龙虾塘DOM的E2∶E3值分别为8.71±0.27和9.34±0.22,与螃蟹塘DOM相比,龙虾塘DOM具有更小的相对分子量。螃蟹塘和龙虾塘DOM的E2∶E3值在高光辐照强度下随时间不断增加。螃蟹塘和龙虾塘DOM的SUVA254值分别为(6.9±0.57)和(5.8±0.25)L/(mg·m),高光强辐照过程中,螃蟹塘和龙虾塘DOM的SUVA254值随辐照时间增加而下降。螃蟹塘和龙虾塘DOM的HIX值分别为3.49±0.39和2.87±0.09,HIX值在低光辐照强度下变化不明显,而高光辐照强度过程中,2个淡水养殖池塘DOM的HIX值均随辐照时间增加呈现上升趋势。螃蟹塘和龙虾塘DOM的BIX值分别为0.9±0.01和0.87±0.01,高光辐照强度下,淡水养殖池塘DOM的BIX值随辐照时间增加均下降。螃蟹塘和龙虾塘DOM的FI值分别为1.63±0.01和1.61±0.01。螃蟹塘和龙虾塘DOM的Peak C/Peak T值分别为1.45±0.02和1.21±0.05,高光辐照强度过程中,2个淡水养殖池塘DOM Peak C/Peak T值均不断上升。
2.3 不同光辐照强度下淡水养殖池塘DOM PPRIs的光化学形成
2.3.1 PPRIs的生成速率
TMP和FFA在水中的光降解遵循着伪一级动力学,具体见附件Ⅱ,而hTPA生成与光辐照时间呈线性关系(图5a,b,c)。低光辐照强度下,螃蟹塘DOM的 、 和R·OH分别为3.87×10-9、4.78×10-9和4.19×10-12 mol/(L·s),龙虾塘DOM的 、 和R·OH分别为1.11×10-9、1.26×10-9和1.76×10-12 mol/(L·s)。高光辐照强度下,螃蟹塘DOM的 、 和R·OH分别为1.41×10-8、1.32×10-8和5.52×10-12 mol/(L·s),龙虾塘DOM的 、 和R·OH分别为9.77×10-9、6.34×10-9和2.50×10-12 mol/(L·s)。不同淡水养殖池塘DOM的PPRIs生成速率存在差异,在相同光辐照强度下,螃蟹塘DOM 3种PPRIs的生成速率均大于龙虾塘DOM(P<0.05)。
2.3.2 PPRIs的稳态浓度
淡水养殖池塘DOM的PPRIs稳态浓度如表2所示。本研究中,低光辐照强度下,螃蟹塘DOM的[3DOM*]ss、[1O2]ss和[·OH]ss分别为7.31×10-16、1.17×10-14和6.34×10-18 mol/L,龙虾塘DOM的[3DOM*]ss、[1O2]ss和[·OH]ss分别为2.09×10-16、0.31×10-14和2.67×10-18 mol/L。高光辐照强度下,螃蟹塘DOM的[3DOM*]ss、[1O2]ss和[·OH]ss分别为26.69×10-16、4.89×10-14和8.36×10-18 mol/L,龙虾塘DOM的[3DOM*]ss、[1O2]ss和[·OH]ss分别为18.44×10-16、2.35×10-14和3.79×10-18 mol/L。光辐照强度越高,淡水养殖池塘DOM的PPRIs稳态浓度越高。同一光辐照强度下,PPRIs的稳态浓度存在异质性,螃蟹塘DOM 3种PPRIs的稳态浓度均高于龙虾塘DOM的PPRIs稳态浓度。
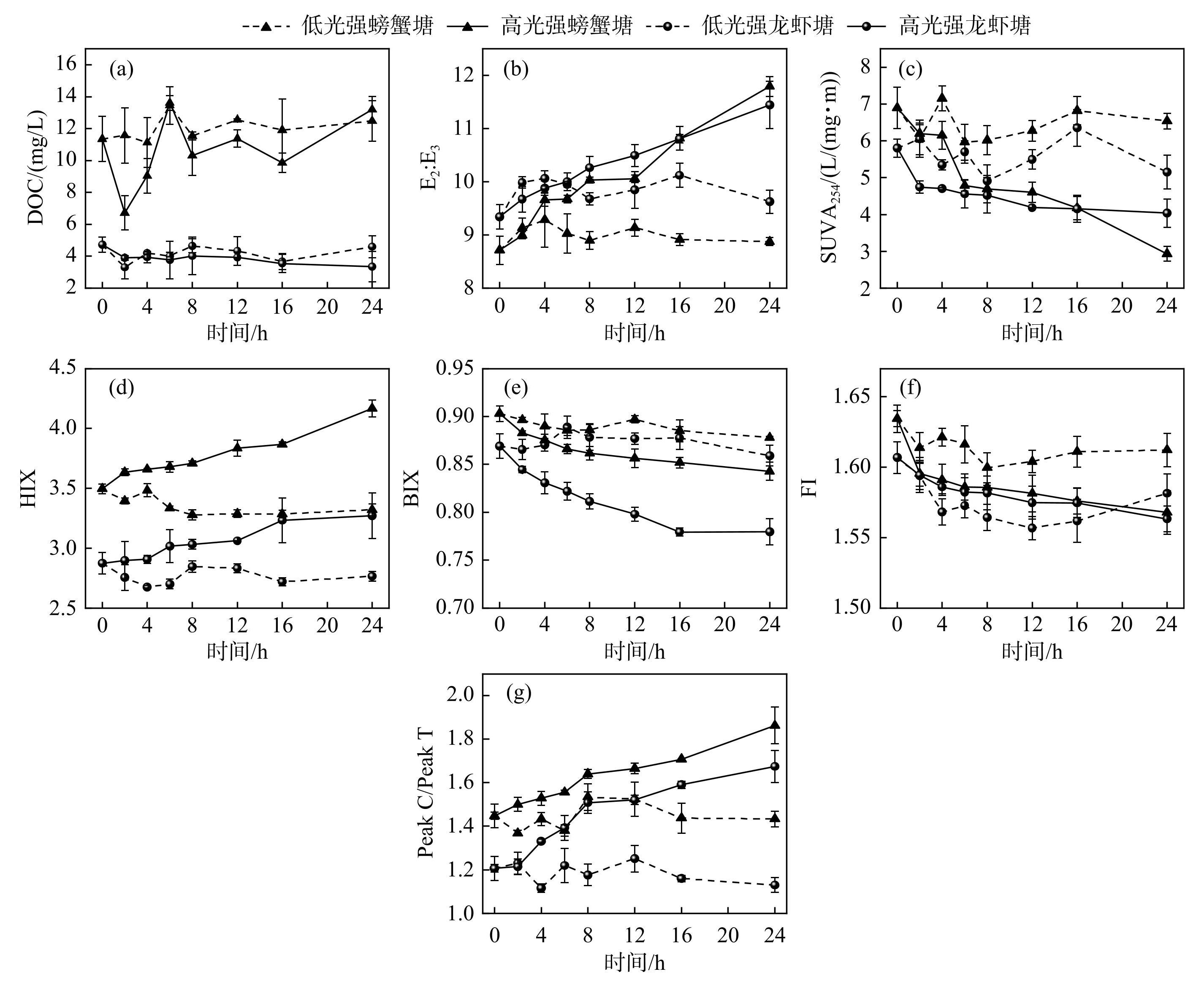
图4淡水养殖池塘光化学过程中DOC浓度和DOM光谱特征参数变化
Fig.4Variation of DOC concentration and DOM spectral characterization parameters during photochemical processes in freshwater aquaculture ponds

图5淡水养殖池塘DOM光化学过程中分子探针变化
Fig.5Changes in molecular probes during photochemistry of DOM in freshwater aquaculture ponds
2.3.3 PPRIs的量子产率
淡水养殖池塘DOM的PPRIs量子产率如表2所示。低光辐照强度下,螃蟹塘DOM的 、 和Φ·OH分别为8.11×10-3、1×10-2和8.76×10-6,龙虾塘DOM的 、 和Φ·OH分别为5.20×10-3、5.89×10-3和8.27×10-6。高光辐照强度下,螃蟹塘DOM的 、 和Φ·OH分别为5.55×10-3、5.18×10-3和2.16×10-6,龙虾塘DOM的 、 和Φ·OH分别为8.20×10-3、5.32×10-3和2.10×10-6。
表2不同光辐照强度下淡水养殖池塘DOM的PPRIs稳态浓度和量子产率
Tab.2 Steady-state concentration and quantum yield of PPRIs in DOM of freshwater aquaculture ponds under different light irradiation intensities
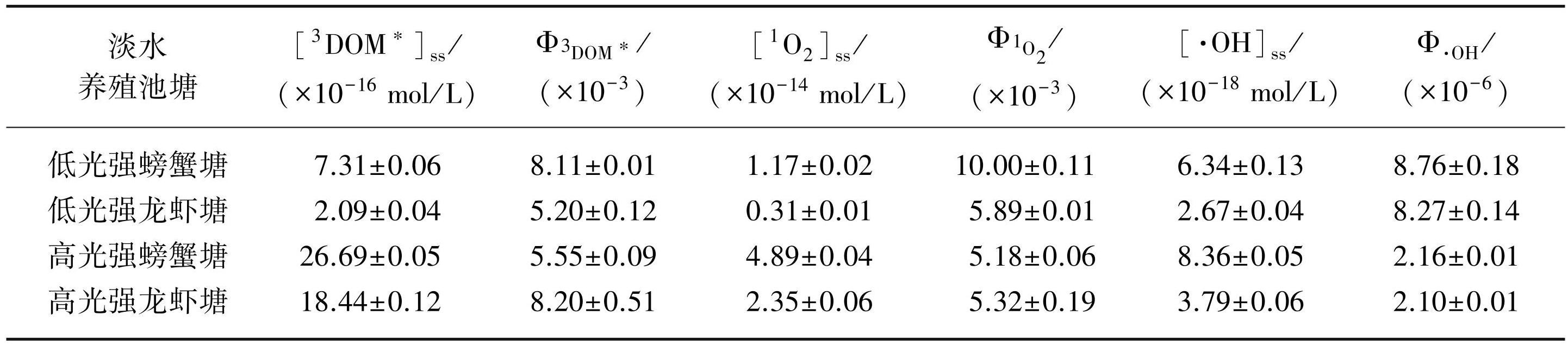
3 讨论
本研究中螃蟹塘和龙虾塘基本水质指标存在一定的差异,螃蟹塘的DOC浓度和富营养化程度更高,但两者pH值相差不大(均约为9),这与淡水虾蟹类更适应碱性较强的水环境有关[50]。由EEMs-PARAFAC所得的Fmax值可以揭示DOM光化学过程荧光强度随时间的变化。对于淡水养殖池塘DOM的荧光组分,螃蟹塘DOM的荧光强度大于龙虾塘DOM,这与DOC结果一致。螃蟹塘和龙虾塘DOM主要成分均为类腐殖质(C1+C2)。由于残余饵料沉积及水产品代谢产物极易被微生物利用[51],所以本研究中微生物代谢的DOM源(C1)贡献较大。光辐照强度是养殖池塘DOM荧光组分光降解的重要影响因素,低光辐照强度下,只有螃蟹塘DOM中C1组分下降,其余组分均没有变化,这主要是由于DOM在低辐照强度下获得的能量不足以破坏DOM的结构。而在高光辐照强度下,淡水养殖池塘DOM各组分的荧光强度均呈现出明显下降趋势,其中类蛋白组分占比最小但光降解潜力最高,这与大型水生植物源和藻源DOM中类蛋白组分表现出更高的降解率结果相一致[52]。
由UV-Vis和EEMs获得的光谱特征参数是表征DOM性质的重要指标。E2∶E3值可以评估DOM的相对分子量大小,其值与相对分子量大小呈反比[53],高光辐照强度下,淡水养殖池塘DOM相对分子量随辐照时间不断减小。有文献报道DOM分子量越小,其生物可利用性越高[54],这说明光辐照使得DOM光化学产物具有更高的生物可利用性。SUVA254值可描述DOM的亲疏水性和芳香性水平,其值越大,DOM的疏水性和芳香性越高,一般认为SUVA254值大于4 L/(mg·m),则DOM属于高芳香性和疏水性物质[55]。淡水养殖池塘DOM的疏水性和芳香性较高且螃蟹塘DOM的疏水性和芳香性更高。高光辐照强度下,淡水养殖池塘DOM疏水性和芳香性随光辐照时间均降低。HIX值可以表征DOM样品的腐殖化程度,HIX值越高,DOM腐殖化程度越高,结构越复杂,一般认为HIX<4时,DOM主要为低腐殖化的生物来源[56]。本文低HIX值反映了淡水养殖池塘DOM腐殖化程度较低,与Wang等[21]报道的我国盐城市淡水养殖池塘DOM腐殖化程度相当(0.40~4.36)。高光强光辐照提高了淡水养殖池塘DOM腐殖化程度,这可能是由于类蛋白组分比类腐殖质组分的光降解速率更高(图3),类腐殖质组分光辐照后相对丰度上升,这与Gu[10]等研究发现水热炭DOM中类蛋白组分光降解速率最高,且腐殖化程度随辐照时间增加而升高的结果一致。一般来说,BIX值用来表征微生物来源DOM的相对贡献程度,其中BIX值在0.8~1.0范围内,则表示DOM属于强自生源组分,BIX值大于1则表示DOM为生物来源组分[57]。淡水养殖池塘DOM属于强自生源组分,DOM的强自生源属性随高光强辐照时间增加而下降。FI值用来表征DOM的来源,当FI<1.4时,DOM以陆源输入为主;当FI>1.9时,DOM以内源输入为主[58]。本研究淡水养殖池塘DOM的FI值(1.61~1.63),与吴峰等[59]报道的我国东营市近海养殖水体DOM的FI值(1.63~1.73)和Wang等[21]报道的我国盐城市淡水养殖池塘DOM的FI值(1.58~1.94)相当,表明养殖池塘DOM兼具陆源与内源属性。本研究采样时间为6月份,采样池塘处于丰水期,降雨和地表径流为淡水养殖池塘DOM的陆源输入源,而浮游生物和藻类的活动、剩余饲料颗粒的浸出以及水产品排泄物属于内源输入,这可能是DOM兼具陆源和内源的主要原因[6]。Peak C/Peak T值表示惰性类腐殖质物质与不稳定类蛋白组分荧光强度比值,其值越大,表明DOM惰性组分占比越高[60],螃蟹塘DOM与龙虾塘DOM的Peak C/Peak T值均大于1,说明类腐殖质物质占比高于类蛋白物质,与三维荧光数据表明的C1和C2组分占比较高一致,其值随高光强光辐照时间的增加而上升,表明高强度光辐照有效地促进了DOM易光解组分的降解。
除了荧光组分的变化,淡水养殖水体DOM光化学过程还伴随着PPRIs的产生。TMP和FFA浓度随着辐照时间的增加而降低,hTPA浓度随着辐照时间的增加而增加,这表明在太阳光辐照过程中,3DOM*、1O2和·OH有效形成。陆源类腐殖质和类蛋白组分可有效促进PPRIs的光化学形成[25],本研究中螃蟹塘DOM 3种PPRIs的生成速率均高于龙虾塘DOM,与螃蟹塘DOM的C2和C3组分相对丰度均大于龙虾塘DOM相吻合。Grandbois等[61]研究发现微生物源DOM的 低于陆源DOM的 ,这说明PPRIs生成速率与DOM的来源有关[36,62]。高光辐照强度下,2种养殖池塘DOM的 均高于我国南海市区和河口下游DOM的3DOM*生成速率(0.76×10-9~7.19×10-9 mol/(L·s))[32],R·OH高于我国莱州湾海水养殖区DOM的·OH生成速率约1个数量级[30],Qian等[63]研究也发现淡水R·OH大于海水R·OH,这说明淡水养殖池塘DOM的·OH生成能力大于海水DOM,可能与海水中DOC浓度较低有关[64]。先前的研究表明3DOM*、1O2和·OH在天然水体中的稳态浓度分别为10-15~10-13、10-15~10-13和10-18~10-16 mol/L量级[29,65-67],本研究也有类似的量级(10-15、10-14和10-18 mol/L)。无论光强如何,[1O2]ss比[3DOM*]ss均高一个数量级,这是因为基态DOM会吸收光子能量,经系间窜越形成3DOM*,3DOM*可以通过能量转移敏化水中的溶解氧生成1O2,而在3DOM*定量实验中,只能捕获到还原电位足以氧化TMP的3DOM*[66-67]。Parker等[68]报道了[3DOM*]ss随着卤素离子强度的增加而增加,螃蟹塘F-(0.63 mg/L)、Cl-(65.11 mg/L)和Br-(0.27 mg/L)浓度均高于龙虾塘F-(0.37 mg/L)、Cl-(56.54 mg/L)和Br-(0.24 mg/L)浓度,这可能是螃蟹塘DOM的[3DOM*]ss高于龙虾塘DOM 的重要原因。抗生素是养殖水体典型的污染物,藻毒素在蓝藻水华暴发期间浓度偏高,PPRIs虽瞬时产生,但是氧化性很强,其对养殖水体中抗生素和藻毒素具有光降解作用[16,19,69-70],因而通过DOM光化学反应量化PPRIs的稳态浓度对阐明养殖池塘有机污染物归趋具有重要意义。本研究中,淡水养殖池塘DOM的 、 略低于Wasswa等[71]报道的纽约Adirondack地区湖泊对应的量子产率(1.3×10-2~4.7×10-2和1.5×10-2~3.3×10-2),但Φ·OH处于同一量级(1.4×10-6~12.9×10-6)。Maizel 等[62]研究了不同营养状态湖泊中DOM组成与光化学之间的关系,发现淡水DOM中的高芳香族结构与3DOM*形成速率呈正相关,但与3DOM*量子产率呈负相关,本研究也有类似的现象,高光辐照强度下,尽管螃蟹塘DOM的 大于龙虾塘DOM的 ,但是螃蟹塘DOM的 却小于龙虾塘DOM的 ,这可能是由于DOM芳香性结构越多会导致其溶液吸收系数更高(图6)。
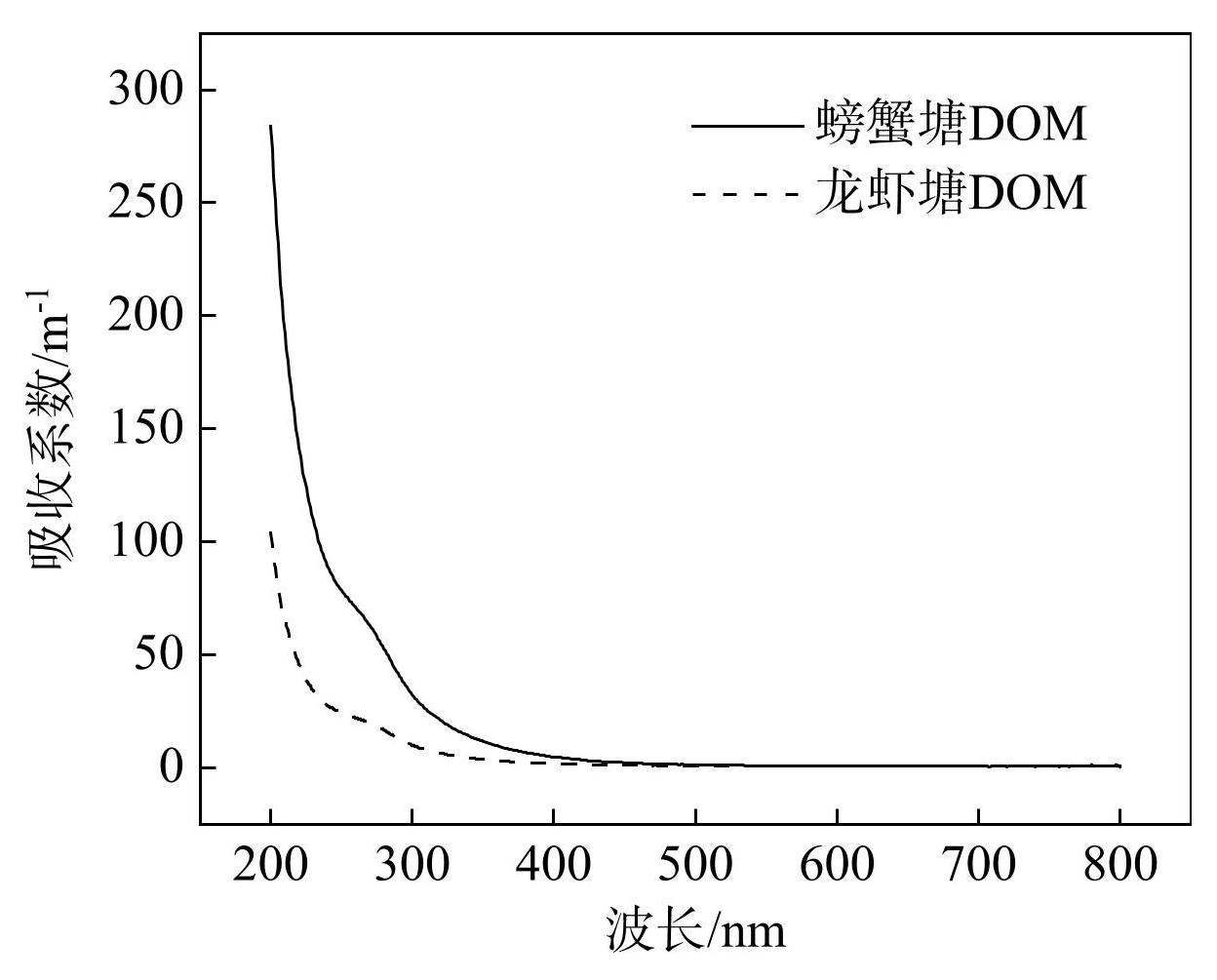
图6淡水养殖池塘DOM溶液吸收系数
Fig.6Absorption coefficient of loose DOM solution in freshwater aquaculture ponds
4 结论
1)淡水养殖池塘DOM可分离出3个荧光组分,包括微生物源类腐殖质组分C1、陆源类腐殖质组分C2和类蛋白组分C3,其中类腐殖质组分的荧光强度占比高(均大于73%),但类蛋白组分具有较高的光化学反应活性。
2)淡水养殖池塘DOM的相对分子量较小、芳香性较高、腐殖化程度较低,其来源受陆源和内源共同影响。光辐照强度对DOM的光化学过程具有重要影响,高光辐照强度下,DOM的荧光组分含量、分子量、芳香性均呈现降低的趋势,而低光辐照强度下DOM自身组分和性质变化不显著。
3)淡水养殖池塘DOM的光化学过程中产生了大量的PPRIs,且PPRIs的生成潜能随着光辐照强度的升高而升高。高光辐照强度下,淡水养殖池塘DOM光化学过程中3DOM*、1O2和·OH生成速率分别为9.77×10-9~1.41×10-8、6.34×10-9~1.32×10-8和2.5×10-12~5.52×10-12 mol/(L·s)。中华绒螯蟹塘DOM具有更高的PPRIs生成潜能。
致谢:采样过程中得到张秀梅老师的帮助,灯源光谱检测得到杜瑛珣和苏雅玲两位老师的支持,在此表示诚挚的谢意!
5 附录
附图Ⅰ、附表Ⅰ~Ⅱ、附件Ⅰ~Ⅱ见电子版(DOI: 10.18307/2025.0222)。

图1由PARAFAC解析养殖池塘DOM EEMs所得荧光组分及对应荷载
Fig.1Analysis of fluorescence fractions and corresponding loads obtained from DOM EEMs in aquaculture ponds by PARAFAC
下载:
全尺寸图片
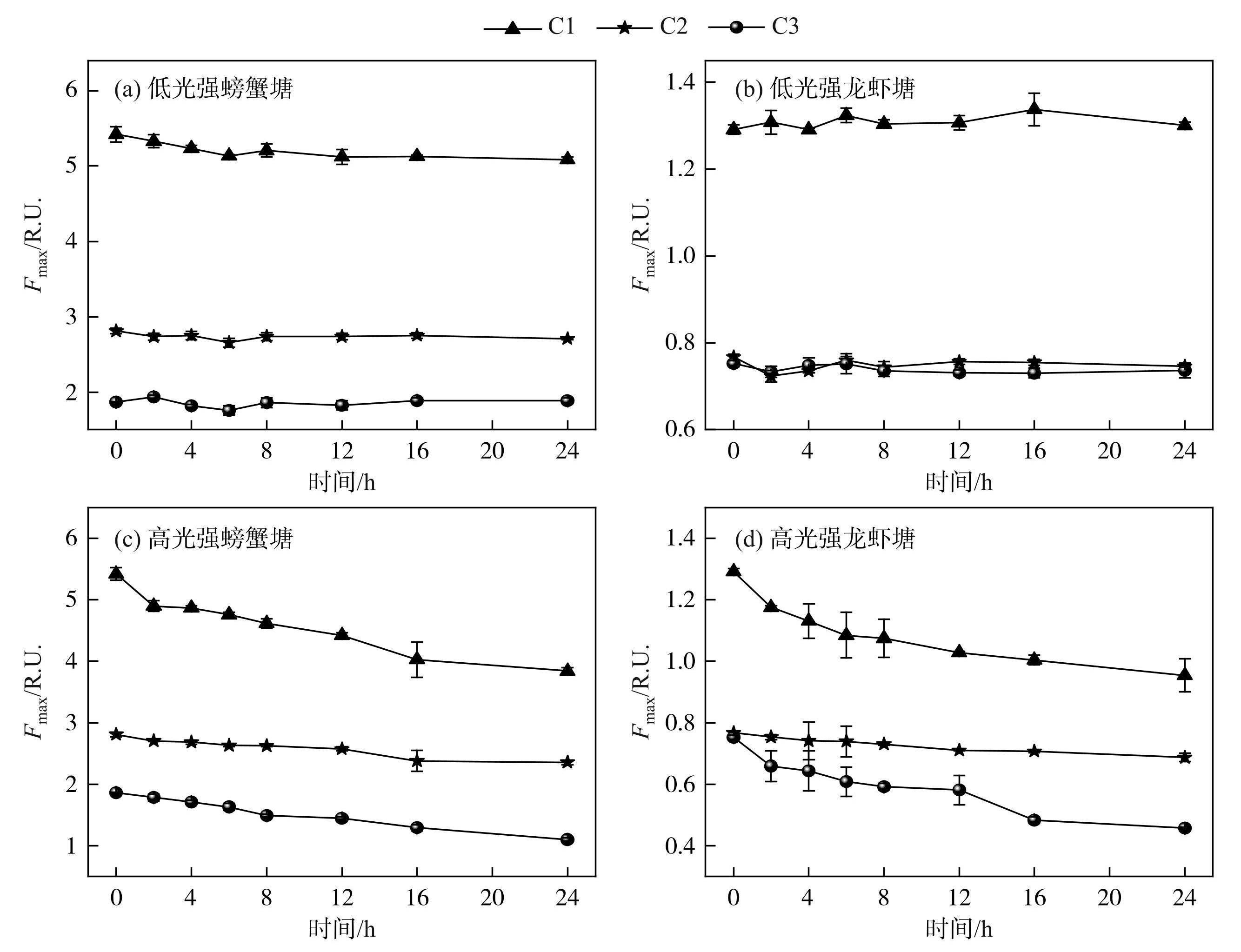
图2淡水养殖池塘DOM光转化过程中最大荧光强度变化
Fig.2Changes in maxmum fluorescence intensity during photoconversion of DOM in freshwater aquaculture ponds
下载:
全尺寸图片

图3淡水养殖池塘DOM光化学过程中荧光强度光化学降解一级动力学(a和b)和荧光强度相对丰度变化(c)
Fig.3First-order kinetics of photochemical degradation of fluorescence intensity (a and b) and changes in relative abundance of fluorescence intensity (c) during photoconversion of DOM in freshwater aquaculture ponds
下载:
全尺寸图片
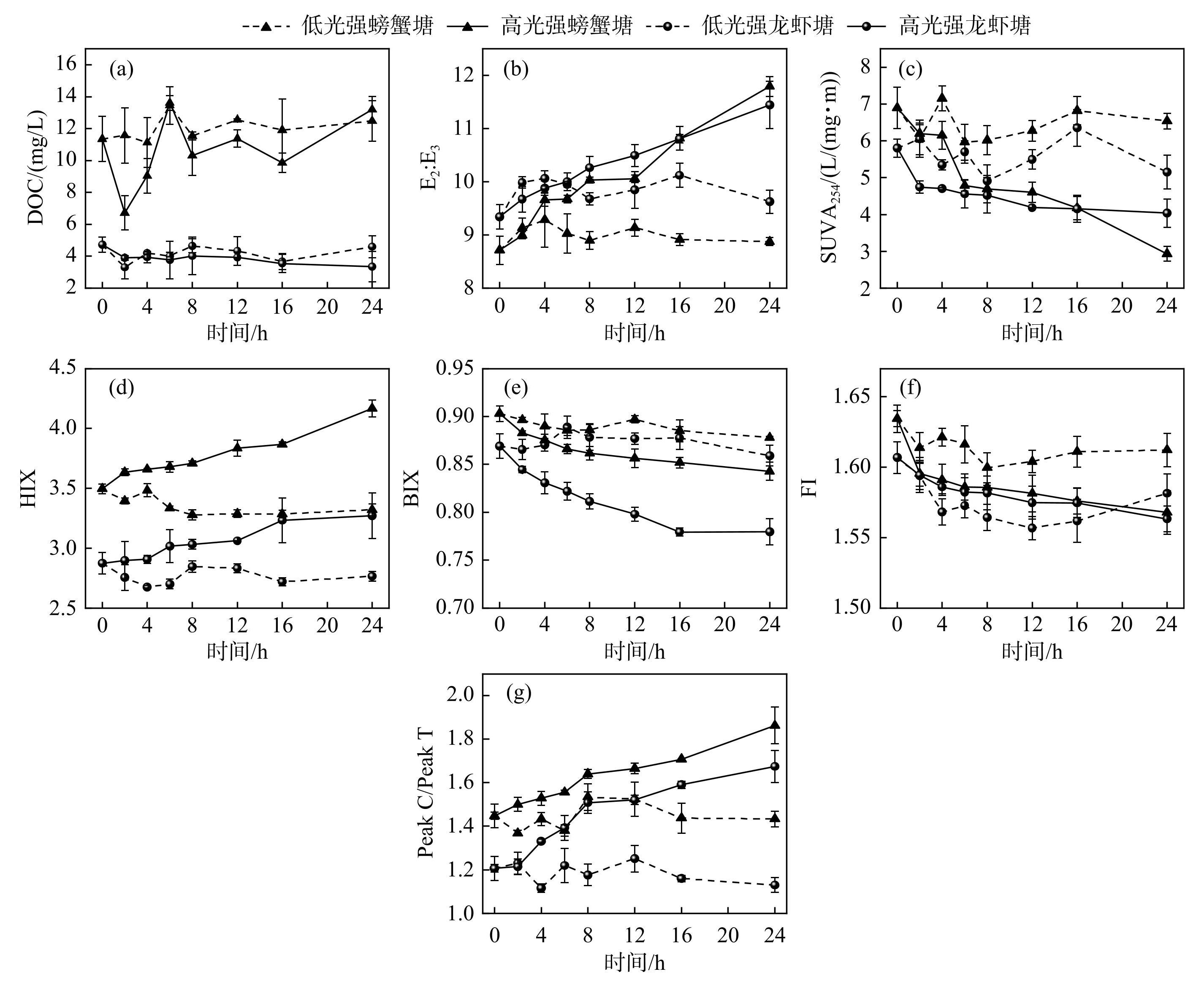
图4淡水养殖池塘光化学过程中DOC浓度和DOM光谱特征参数变化
Fig.4Variation of DOC concentration and DOM spectral characterization parameters during photochemical processes in freshwater aquaculture ponds
下载:
全尺寸图片

图5淡水养殖池塘DOM光化学过程中分子探针变化
Fig.5Changes in molecular probes during photochemistry of DOM in freshwater aquaculture ponds
下载:
全尺寸图片
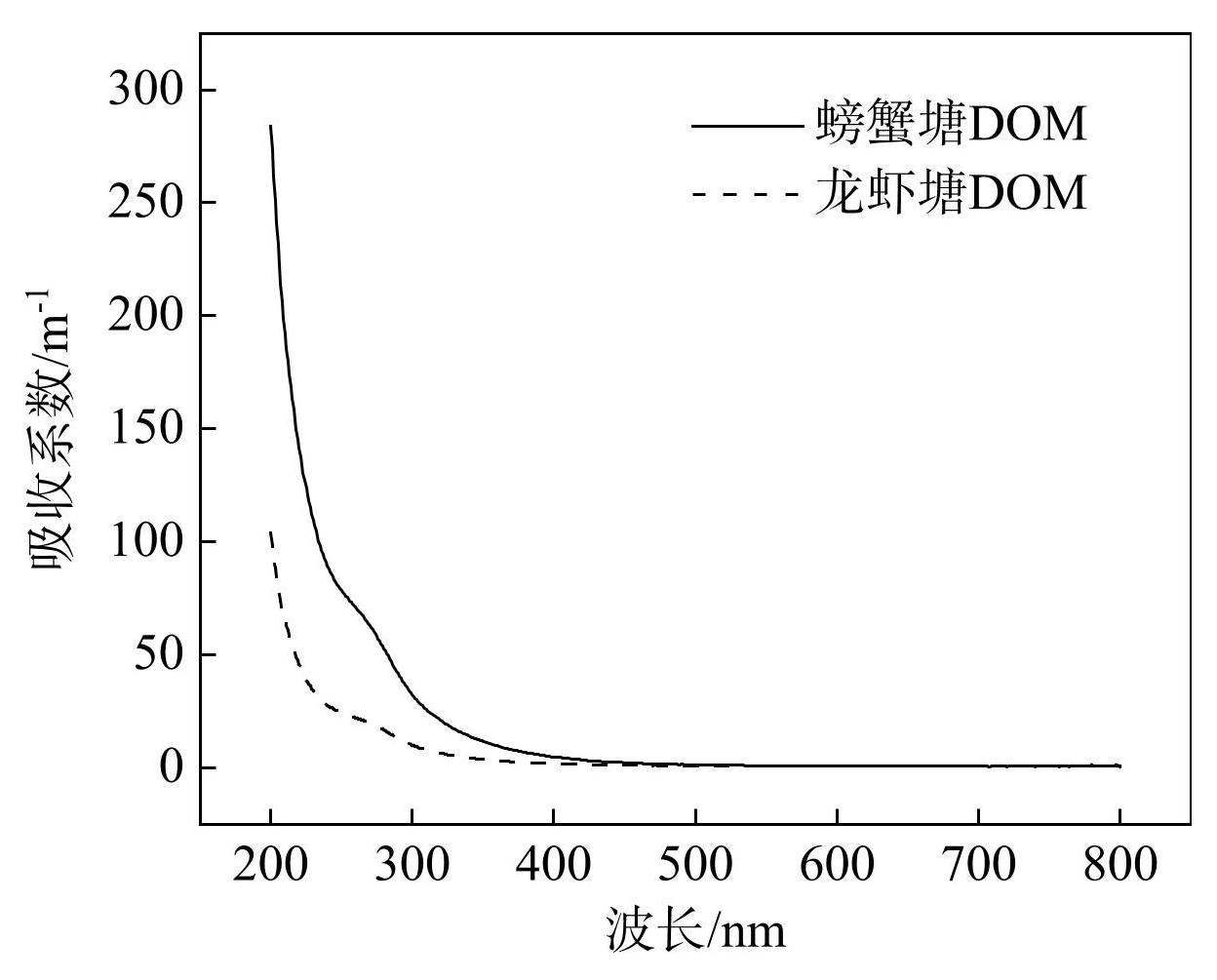
图6淡水养殖池塘DOM溶液吸收系数
Fig.6Absorption coefficient of loose DOM solution in freshwater aquaculture ponds
下载:
全尺寸图片

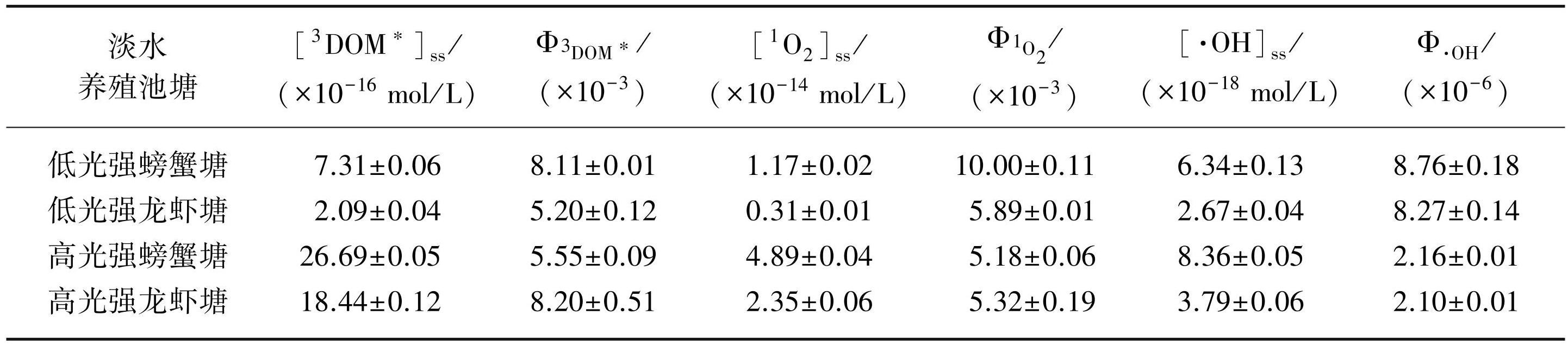
[1] |
He C, He D, Chen CM et al. Application of Fourier transform ion cyclotron resonance mass spectrometry in the composition analysis of dissolved organic matter. Scientia Sinica Terrae,2022,52(12):2323-2341. DOI:10.1360/SSTe-2021-0390.[何晨, 何丁, 陈春茂等. 傅里叶变换离子回旋共振质谱在溶解性有机质组成分析中的应用. 中国科学: 地球科学,2022,52(12):2323-2341.]
|
[2] |
Artifon V, Zanardi-Lamardo E, Fillmann G. Aquatic organic matter: Classification and interaction with organic microcontaminants. Science of the Total Environment,2019,649:1620-1635. DOI:10.1016/j.scitotenv.2018.08.385.
|
[3] |
Yamada N, Katoh M. Feature of lead complexed with dissolved organic matter on lead immobilization by hydroxyapatite in aqueous solutions and soils. Chemosphere,2020,249:126122. DOI:10.1016/j.chemosphere.2020.126122.
|
[4] |
Minor EC, Swenson MM, Mattson BM et al. Structural characterization of dissolved organic matter: A review of current techniques for isolation and analysis. Environmental Science Processes & Impacts,2014,16(9):2064-2079. DOI:10.1039/c4em00062e.
|
[5] |
Ma YC, Sun LY, Liu CY et al. A comparison of methane and nitrous oxide emissions from inland mixed-fish and crab aquaculture ponds. Science of the Total Environment,2018,637/638:517-523. DOI:10.1016/j.scitotenv.2018.05.040.
|
[6] |
Holmer M. Environmental issues of fish farming in offshore waters: Perspectives,concerns and research needs. Aquaculture Environment Interactions,2010,1(1):57-70. DOI:10.3354/aei00007.
|
[7] |
Dutta Majumdar R, Bliumkin L, Lane D et al. Analysis of DOM phototransformation using a looped NMR system integrated with a sunlight simulator. Water Research,2017,120:64-76. DOI:10.1016/j.watres.2017.04.067.
|
[8] |
Engel M, Chefetz B. Removal of triazine-based pollutants from water by carbon nanotubes: Impact of dissolved organic matter(DOM)and solution chemistry. Water Research,2016,106:146-154. DOI:10.1016/j.watres.2016.09.051.
|
[9] |
Zhang T, Ma H, Hong ZC et al. Photo-reactivity and photo-transformation of algal dissolved organic matter unraveled by optical spectroscopy and high-resolution mass spectrometry analysis. Environmental Science & Technology,2022,56(18):13439-13448. DOI:10.1021/acs.est.2c03524.
|
[10] |
Gu XC, Chen BF, Liu H et al. Photochemical behavior of dissolved organic matter derived from Alternanthera philoxeroides hydrochar: Insights from molecular transformation and photochemically reactive intermediates. Journal of Hazardous Materials,2024,461:132591. DOI:10.1016/j.jhazmat.2023.132591.
|
[11] |
Obernosterer I, Reitner B, Herndl GJ. Contrasting effects of solar radiation on dissolved organic matter and its bioavailability to marine bacterioplankton. Limnology and Oceanography,1999,44(7):1645-1654. DOI:10.4319/lo.1999.44.7.1645.
|
[12] |
Neilen AD, Hawker DW, O'Brien KR et al. Phytotoxic effects of terrestrial dissolved organic matter on a freshwater cyanobacteria and green algae species is affected by plant source and DOM chemical composition. Chemosphere,2017,184:969-980. DOI:10.1016/j.chemosphere.2017.06.063.
|
[13] |
Haag WR, Hoigné J. Photo-sensitized oxidation in natural water via ·OH radicals. Chemosphere,1985,14(11/12):1659-1671. DOI:10.1016/0045-6535(85)90107-9.
|
[14] |
Page SE, Logan JR, Cory RM et al. Evidence for dissolved organic matter as the primary source and sink of photochemically produced hydroxyl radical in Arctic surface waters. Environmental Science Processes & Impacts,2014,16(4):807-822. DOI:10.1039/c3em00596h.
|
[15] |
Song N, Wu DG, Xu HC et al. Integrated evaluation of the reactive oxygen species(ROS)production characteristics in one large lake under alternating flood and drought conditions. Water Research,2022,225:119136. DOI:10.1016/j.watres.2022.119136.
|
[16] |
Wang JQ, Chen JW, Qiao XL et al. DOM from mariculture ponds exhibits higher reactivity on photodegradation of sulfonamide antibiotics than from offshore seawaters. Water Research,2018,144:365-372. DOI:10.1016/j.watres.2018.07.043.
|
[17] |
Guerard JJ, Chin YP, Mash H et al. Photochemical fate of sulfadimethoxine in aquaculture waters. Environmental Science & Technology,2009,43(22):8587-8592. DOI:10.1021/es9020537.
|
[18] |
Guerard JJ, Chin YP. Photodegradation of ormetoprim in aquaculture and stream-derived dissolved organic matter. Journal of Agricultural and Food Chemistry,2012,60(39):9801-9806. DOI:10.1021/jf302564d.
|
[19] |
An JQ, Jiang YC, Cao HF et al. Photodegradation of glyphosate in water and stimulation of by-products on algae growth. Ecotoxicology and Environmental Safety,2023,263:115211. DOI:10.1016/j.ecoenv.2023.115211.
|
[20] |
He YY, Häder DP. UV-B-induced formation of reactive oxygen species and oxidative damage of the cyanobacterium Anabaena sp.: Protective effects of ascorbic acid and N-acetyl-L-cysteine. Journal of Photochemistry and Photobiology B, Biology,2002,66(2):115-124. DOI:10.1016/s1011-1344(02)00231-2.
|
[21] |
Wang DD, Song CT, Zhang BL et al. Deciphering dissolved organic matter from freshwater aquaculture ponds in eastern China based on optical and molecular signatures. Process Safety and Environmental Protection,2021,155:122-130. DOI:10.1016/j.psep.2021.09.025.
|
[22] |
Ryan KA, Palacios LC, Encina F et al. Assessing inputs of aquaculture-derived nutrients to streams using dissolved organic matter fluorescence. Science of the Total Environment,2022,807(Pt 2):150785. DOI:10.1016/j.scitotenv.2021.150785.
|
[23] |
Xu HC, Zou L, Guan DX et al. Molecular weight-dependent spectral and metal binding properties of sediment dissolved organic matter from different origins. Science of the Total Environment,2019,665:828-835. DOI:10.1016/j.scitotenv.2019.02.186.
|
[24] |
Huguet A, Vacher L, Relexans S et al. Properties of fluorescent dissolved organic matter in the Gironde Estuary. Organic Geochemistry,2009,40(6):706-719. DOI:10.1016/j.orggeochem.2009.03.002.
|
[25] |
Ayeni TT, Iwamoto Y, Takeda K et al. Optical properties of dissolved organic matter in Japanese rivers and contributions to photoformation of reactive oxygen species. Science of the Total Environment,2022,826:153671. DOI:10.1016/j.scitotenv.2022.153671.
|
[26] |
Nosaka Y, Takahashi S, Sakamoto H et al. Reaction mechanism of Cu(II)-grafted visible-light responsive TiO2 and WO3 photocatalysts studied by means of ESR spectroscopy and chemiluminescence photometry. Journal of Physical Chemistry C,2011,115(43):21283-21290. DOI:10.1021/jp2070634.
|
[27] |
Chen TT, Hu YH, Cen Y et al. A dual-emission fluorescent nano complex of gold-cluster-decorated silica particles for live cell imaging of highly reactive oxygen species. Journal of the American Chemical Society,2013,135(31):11595-11602. DOI:10.1021/ja4035939.
|
[28] |
Rosario-Ortiz FL, Canonica S. Probe compounds to assess the photochemical activity of dissolved organic matter. Environmental Science & Technology,2016,50(23):12532-12547. DOI:10.1021/acs.est.6b02776.
|
[29] |
Vione D, Minella M, Maurino V et al. Indirect photochemistry in sunlit surface waters: Photoinduced production of reactive transient species. Chemistry,2014,20(34):10590-10606. DOI:10.1002/chem.201400413.
|
[30] |
Liu Y, Qiao XL, Chen YB et al. Photochemical activity of dissovled organic matter in the effluent from mariculture areas. Environmental Chemistry.2016,35(8):1611-1618. DOI:10.7524/j.issn.0254-6108.[刘远, 乔显亮, 陈一波等. 海水养殖区排水中溶解性有机质的光化学研究. 环境化学,2016,35(8):1611-1618.]
|
[31] |
Wang X, Yuan L, Chen Y. Research on the structure-activity relationship of photochemical activity of dissolved organic matter in lake waters. Journal of Huazhong University of Science and Technology: Natural Science Edition,2023,51(7):1-8. DOI:10.13245/j.hust.240276.[王兴, 员乐, 陈勇. 湖泊水体溶解性有机物光化学活性构效关系研究. 华中科技大学学报: 自然科学版,2023,51(7):1-8.]
|
[32] |
Liao ZY, Wang Y, Xie KT et al. Photochemistry of dissolved organic matter in water from the Pearl River(China): Seasonal patterns and predictive modelling. Water Research,2022,208:117875. DOI:10.1016/j.watres.2021.117875.
|
[33] |
Jiang HY, Zhao MZ, Hong WJ et al. Mechanistic and kinetic consideration of the photochemically generated oxidative organic radicals in dissolved black carbon solutions under simulated solar irradiation. Environmental Science & Technology,2024,58(1):760-770. DOI:10.1021/acs.est.3c07216.
|
[34] |
Hou JY, Zhang RC, Ge JF et al. Molecular and optical signatures of photochemical transformation of dissolved organic matter: Nonnegligible role of suspended particulate matter in urban river. Science of the Total Environment,2023,903:166842. DOI:10.1016/j.scitotenv.2023.166842.
|
[35] |
Page SE, Arnold WA, McNeill K. Terephthalate as a probe for photochemically generated hydroxyl radical. Journal of Environmental Monitoring: JEM,2010,12(9):1658-1665. DOI:10.1039/c0em00160k.
|
[36] |
Timko SA, Romera-Castillo C, Jaffé R et al. Photo-reactivity of natural dissolved organic matter from fresh to marine waters in the Florida Everglades, USA. Environmental Science: Processes & Impacts,2014,16(4):866-878. DOI:10.1039/C3EM00591G.
|
[37] |
Murphy KR, Stedmon CA, Graeber D et al. Fluorescence spectroscopy and multi-way techniques. PARAFAC. Analytical Methods,2013,5(23):6557-6566. DOI:10.1039/C3AY41160E.
|
[38] |
Stedmon CA, Bro R. Characterizing dissolved organic matter fluorescence with parallel factor analysis: A tutorial. Limnology and Oceanography: Methods,2008,6(11):572-579. DOI:10.4319/lom.2008.6.572.
|
[39] |
Ge MT, Wang BY, Chen BF et al. Hydrochar and its dissolved organic matter aged in a 30-month rice-wheat rotation system: Do primary aging factors alter at different stages?Environmental Science & Technology,2024,58(6):3019-3030. DOI:10.1021/acs.est.3c08044.
|
[40] |
Batista-Andrade JA, Diaz E, Iglesias Vega D et al. Spatiotemporal analysis of fluorescent dissolved organic matter to identify the impacts of failing sewer infrastructure in urban streams. Water Research,2023,229:119521. DOI:10.1016/j.watres.2022.119521.
|
[41] |
Tran NH, Ngo HH, Urase T et al. A critical review on characterization strategies of organic matter for wastewater and water treatment processes. Bioresource Technology,2015,193:523-533. DOI:10.1016/j.biortech.2015.06.091.
|
[42] |
Yamashita Y, Boyer JN, Jaffé R. Evaluating the distribution of terrestrial dissolved organic matter in a complex coastal ecosystem using fluorescence spectroscopy. Continental Shelf Research,2013,66:136-144. DOI:10.1016/j.csr.2013.06.010.
|
[43] |
Gao ZY, Guéguen C. Size distribution of absorbing and fluorescing DOM in Beaufort Sea, Canada Basin. Deep Sea Research Part I: Oceanographic Research Papers,2017,121:30-37. DOI:10.1016/j.dsr.2016.12.014.
|
[44] |
Vines M, Terry LG. Evaluation of the biodegradability of fluorescent dissolved organic matter via biological filtration. AWWA Water Science,2020,2(5):e1201. DOI:10.1002/aws2.1201.
|
[45] |
Yamashita Y, Panton A, Mahaffey C et al. Assessing the spatial and temporal variability of dissolved organic matter in Liverpool Bay using excitation-emission matrix fluorescence and parallel factor analysis. Ocean Dynamics,2011,61(5):569-579. DOI:10.1007/s10236-010-0365-4.
|
[46] |
Shakil S, Tank SE, Kokelj SV et al. Particulate dominance of organic carbon mobilization from thaw slumps on the Peel Plateau, NT: Quantification and implications for stream systems and permafrost carbon release. Environmental Research Letters,2020,15(11):114019. DOI:10.1088/1748-9326/abac36.
|
[47] |
Kim J, Kim Y, Park SE et al. Impact of aquaculture on distribution of dissolved organic matter in coastal Jeju Island, Korea,based on absorption and fluorescence spectroscopy. Environmental Science and Pollution Research,2022,29(1):553-563. DOI:10.1007/s11356-021-15553-3.
|
[48] |
Zhou YL, Martin P, Müller M. Composition and cycling of dissolved organic matter from tropical peatlands of coastal Sarawak, Borneo,revealed by fluorescence spectroscopy and parallel factor analysis. Biogeosciences,2019,16(13):2733-2749. DOI:10.5194/bg-16-2733-2019.
|
[49] |
Berggren M, Gudasz C, Guillemette F et al. Systematic microbial production of optically active dissolved organic matter in subarctic lake water. Limnology and Oceanography,2020,65(5):951-961. DOI:10.1002/lno.11362.
|
[50] |
Figueiró CSM, Bastos de Oliveira D, Russo MR et al. Fish farming water quality monitored by optical analysis: The potential application of UV-Vis absorption and fluorescence spectroscopy. Aquaculture,2018,490:91-97. DOI:10.1016/j.aquaculture.2018.02.027.
|
[51] |
Brisco S, Ziegler S. Effects of solar radiation on the utilization of dissolved organic matter(DOM)from two headwater streams. Aquatic Microbial Ecology,2004,37:197-208. DOI:10.3354/ame037197.
|
[52] |
Fu XW, Du HY, Xu HC. Comparison in UV-induced photodegradation properties of dissolved organic matters with different origins. Chemosphere,2021,280:130633. DOI:10.1016/j.chemosphere.2021.130633.
|
[53] |
Helms JR, Stubbins A, Ritchie JD et al. Absorption spectral slopes and slope ratios as indicators of molecular weight,source,and photobleaching of chromophoric dissolved organic matter. Limnology and Oceanography,2008,53(3):955-969. DOI:10.4319/lo.2008.53.3.0955.
|
[54] |
Nalven SG, Ward CP, Payet JP et al. Experimental metatranscriptomics reveals the costs and benefits of dissolved organic matter photo-alteration for freshwater microbes. Environmental Microbiology,2020,22(8):3505-3521. DOI:10.1111/1462-2920.15121.
|
[55] |
Du YX, Zhang YY, Chen FZ et al. Photochemical reactivities of dissolved organic matter(DOM)in a sub-alpine lake revealed by EEM-PARAFAC: An insight into the fate of allochthonous DOM in alpine lakes affected by climate change. Science of the Total Environment,2016,568:216-225. DOI:10.1016/j.scitotenv.2016.06.036.
|
[56] |
Liu RD, Gu XC, Chen J et al. Spectral characteristics of dissolved organic matter in typical heavy polluted water during dry season and its relationship with water quality. Jiangsu Journal of Agricultural Sciences,2023,39(7):1510-1518. DOI:10.3969/j.issn.1000-4440.[刘润东, 顾鑫才, 陈重军等. 典型重污染水体枯水期溶解性有机质光谱特征及其与水质的相关性. 江苏农业学报,2023,39(7):1510-1518.]
|
[57] |
Li YZ, Zhang YB, Li Z et al. Characterization of colored dissolved organic matter in the northeastern South China Sea using EEMs-PARAFAC and absorption spectroscopy. Journal of Sea Research,2022,180:102159. DOI:10.1016/j.seares.2021.102159.
|
[58] |
Chen ML, Price RM, Yamashita Y et al. Comparative study of dissolved organic matter from groundwater and surface water in the Florida coastal Everglades using multi-dimensional spectrofluorometry combined with multivariate statistics. Applied Geochemistry,2010,25(6):872-880. DOI:10.1016/j.apgeochem.2010.03.005.
|
[59] |
Wu F, Song XX, Chi LB et al. Fluorescence characteristics and environmental indicators of dissolved organic matter in offshore aquaculture waters. Oceanologia et Limnologia Sinica,2022,53(3):597-606. DOI:10.11693/hyhz20211100272.[吴峰, 宋秀贤, 池连宝等. 近海养殖水体溶解性有机质的荧光特征及环境指示意义. 海洋与湖沼,2022,53(3):597-606.]
|
[60] |
Baker A. Fluorescence excitation-emission matrix characterization of some sewage-impacted rivers. Environmental Science & Technology,2001,35(5):948-953. DOI:10.1021/es000177t.
|
[61] |
Grandbois M, Latch DE, McNeill K. Microheterogeneous concentrations of singlet oxygen in natural organic matter isolate solutions. Environmental Science & Technology,2008,42(24):9184-9190. DOI:10.1021/es8017094.
|
[62] |
Maizel AC, Li J, Remucal CK. Relationships between dissolved organic matter composition and photochemistry in lakes of diverse trophic status. Environmental Science & Technology,2017,51(17):9624-9632. DOI:10.1021/acs.est.7b01270.
|
[63] |
Qian JG, Mopper K, Kieber DJ. Photochemical production of the hydroxyl radical in Antarctic waters. Deep Sea Research Part I: Oceanographic Research Papers,2001,48(3):741-759. DOI:10.1016/s0967-0637(00)00068-6.
|
[64] |
Xu HC, Guo LD. Molecular size-dependent abundance and composition of dissolved organic matter in river,lake and sea waters. Water Research,2017,117:115-126. DOI:10.1016/j.watres.2017.04.006.
|
[65] |
Zepp RG, Schlotzhauer PF, Sink RM. Photosensitized transformations involving electronic energy transfer in natural waters: Role of humic substances. Environmental Science & Technology,1985,19(1):74-81. DOI:10.1021/es00131a008.
|
[66] |
Ossola R, Jönsson OM, Moor K et al. Singlet oxygen quantum yields in environmental waters. Chemical Reviews,2021,121(7):4100-4146. DOI:10.1021/acs.chemrev.0c00781.
|
[67] |
Zeng Y, Fang GD, Fu QL et al. Photochemical characterization of paddy water during rice cultivation: Formation of reactive intermediates for As(III)oxidation. Water Research,2021,206:117721. DOI:10.1016/j.watres.2021.117721.
|
[68] |
Parker KM, Pignatello JJ, Mitch WA. Influence of ionic strength on triplet-state natural organic matter loss by energy transfer and electron transfer pathways. Environmental Science & Technology,2013,47(19):10987-10994. DOI:10.1021/es401900j.
|
[69] |
Liu SK, Cui ZG, Ding DS et al. Effect of the molecular weight of DOM on the indirect photodegradation of fluoroquinolone antibiotics. Journal of Environmental Management,2023,348:119192. DOI:10.1016/j.jenvman.2023.119192.
|
[70] |
Sun QY, Zhang TF, Wang FF et al. Ultraviolet photosensitized transformation mechanism of microcystin-LR by natural organic matter in raw water. Chemosphere,2018,209:96-103. DOI:10.1016/j.chemosphere.2018.06.091.
|
[71] |
Wasswa J, Driscoll CT, Zeng T. Photochemical characterization of surface waters from lakes in the adirondack region of New York. Environmental Science & Technology,2020,54(17):10654-10667. DOI:10.1021/acs.est.0c02811.
|